Survivin regulates intracellular stiffness and extracellular matrix production in vascular smooth muscle cells
- PMID: 37868708
- PMCID: PMC10590228
- DOI: 10.1063/5.0157549
Survivin regulates intracellular stiffness and extracellular matrix production in vascular smooth muscle cells
Abstract
Vascular dysfunction is a common cause of cardiovascular diseases characterized by the narrowing and stiffening of arteries, such as atherosclerosis, restenosis, and hypertension. Arterial narrowing results from the aberrant proliferation of vascular smooth muscle cells (VSMCs) and their increased synthesis and deposition of extracellular matrix (ECM) proteins. These, in turn, are modulated by arterial stiffness, but the mechanism for this is not fully understood. We found that survivin is an important regulator of stiffness-mediated ECM synthesis and intracellular stiffness in VSMCs. Whole-transcriptome analysis and cell culture experiments showed that survivin expression is upregulated in injured femoral arteries in mice and in human VSMCs cultured on stiff fibronectin-coated hydrogels. Suppressed expression of survivin in human VSMCs significantly decreased the stiffness-mediated expression of ECM components related to arterial stiffening, such as collagen-I, fibronectin, and lysyl oxidase. By contrast, expression of these ECM proteins was rescued by ectopic expression of survivin in human VSMCs cultured on soft hydrogels. Interestingly, atomic force microscopy analysis showed that suppressed or ectopic expression of survivin decreases or increases intracellular stiffness, respectively. Furthermore, we observed that inhibiting Rac and Rho reduces survivin expression, elucidating a mechanical pathway connecting intracellular tension, mediated by Rac and Rho, to survivin induction. Finally, we found that survivin inhibition decreases FAK phosphorylation, indicating that survivin-dependent intracellular tension feeds back to maintain signaling through FAK. These findings suggest a novel mechanism by which survivin potentially modulates arterial stiffness.
© 2023 Author(s).
Conflict of interest statement
The authors have no conflicts to disclose.
Figures
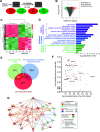
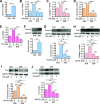
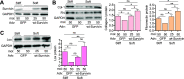
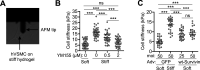
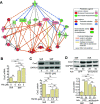
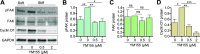
Similar articles
-
Survivin as a mediator of stiffness-induced cell cycle progression and proliferation of vascular smooth muscle cells.APL Bioeng. 2023 Oct 30;7(4):046108. doi: 10.1063/5.0150532. eCollection 2023 Dec. APL Bioeng. 2023. PMID: 37915752 Free PMC article.
-
Mining the Stiffness-Sensitive Transcriptome in Human Vascular Smooth Muscle Cells Identifies Long Noncoding RNA Stiffness Regulators.Arterioscler Thromb Vasc Biol. 2018 Jan;38(1):164-173. doi: 10.1161/ATVBAHA.117.310237. Epub 2017 Oct 19. Arterioscler Thromb Vasc Biol. 2018. PMID: 29051139 Free PMC article.
-
Echinatin maintains glutathione homeostasis in vascular smooth muscle cells to protect against matrix remodeling and arterial stiffening.Matrix Biol. 2023 May;119:1-18. doi: 10.1016/j.matbio.2023.03.007. Epub 2023 Mar 22. Matrix Biol. 2023. PMID: 36958467
-
Vascular Smooth Muscle Cells and Arterial Stiffening: Relevance in Development, Aging, and Disease.Physiol Rev. 2017 Oct 1;97(4):1555-1617. doi: 10.1152/physrev.00003.2017. Physiol Rev. 2017. PMID: 28954852 Review.
-
Simple Models of Complex Mechanics for Improved Hypertension Care: Learning to De-stiffen Arteries.Artery Res. 2023;29(3):94-100. doi: 10.1007/s44200-023-00037-1. Epub 2023 Jul 25. Artery Res. 2023. PMID: 37674758 Free PMC article. Review.
Cited by
-
Transcriptomic and Multi-scale Network Analyses Reveal Key Drivers of Cardiovascular Disease.bioRxiv [Preprint]. 2024 Sep 16:2024.09.11.612437. doi: 10.1101/2024.09.11.612437. bioRxiv. 2024. PMID: 39345636 Free PMC article. Preprint.
References
Grants and funding
LinkOut - more resources
Full Text Sources
Miscellaneous