Adiponectin in the mammalian host influences ticks' acquisition of the Lyme disease pathogen Borrelia
- PMID: 37862360
- PMCID: PMC10619873
- DOI: 10.1371/journal.pbio.3002331
Adiponectin in the mammalian host influences ticks' acquisition of the Lyme disease pathogen Borrelia
Abstract
Arthropod-borne pathogens cause some of the most important human and animal infectious diseases. Many vectors acquire or transmit pathogens through the process of blood feeding. Here, we report adiponectin, the most abundant adipocyte-derived hormone circulating in human blood, directly or indirectly inhibits acquisition of the Lyme disease agent, Borrelia burgdorferi, by Ixodes scapularis ticks. Rather than altering tick feeding or spirochete viability, adiponectin or its associated factors induces host histamine release when the tick feeds, which leads to vascular leakage, infiltration of neutrophils and macrophages, and inflammation at the bite site. Consistent with this, adiponectin-deficient mice have diminished pro-inflammatory responses, including interleukin (IL)-12 and IL-1β, following a tick bite, compared with wild-type animals. All these factors mediated by adiponectin or associated factors influence B. burgdorferi survival at the tick bite site. These results suggest a host adipocyte-derived hormone modulates pathogen acquisition by a blood-feeding arthropod.
Copyright: © 2023 Tang et al. This is an open access article distributed under the terms of the Creative Commons Attribution License, which permits unrestricted use, distribution, and reproduction in any medium, provided the original author and source are credited.
Conflict of interest statement
The authors have declared that no competing interests exist.
Figures
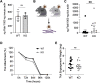
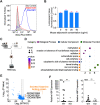
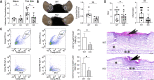
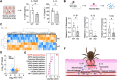
Similar articles
-
The Lyme disease agent co-opts adiponectin receptor-mediated signaling in its arthropod vector.Elife. 2021 Nov 16;10:e72568. doi: 10.7554/eLife.72568. Elife. 2021. PMID: 34783654 Free PMC article.
-
Identification of Lyme borreliae proteins promoting vertebrate host blood-specific spirochete survival in Ixodes scapularis nymphs using artificial feeding chambers.Ticks Tick Borne Dis. 2018 Jul;9(5):1057-1063. doi: 10.1016/j.ttbdis.2018.03.033. Epub 2018 Apr 4. Ticks Tick Borne Dis. 2018. PMID: 29653905 Free PMC article.
-
The abundance of the Lyme disease pathogen Borrelia afzelii declines over time in the tick vector Ixodes ricinus.Parasit Vectors. 2017 May 25;10(1):257. doi: 10.1186/s13071-017-2187-4. Parasit Vectors. 2017. PMID: 28545520 Free PMC article.
-
Vector/host relationships of the Lyme disease spirochete, Borrelia burgdorferi.Rheum Dis Clin North Am. 1989 Nov;15(4):775-87. Rheum Dis Clin North Am. 1989. PMID: 2685930 Review.
-
Pathophysiology of the Lyme disease spirochete, Borrelia burgdorferi, in ixodid ticks.Rev Infect Dis. 1989 Sep-Oct;11 Suppl 6:S1442-50. doi: 10.1093/clinids/11.supplement_6.s1442. Rev Infect Dis. 1989. PMID: 2682956 Review.
References
MeSH terms
Substances
Grants and funding
LinkOut - more resources
Full Text Sources
Medical
Molecular Biology Databases