The plant siRNA landscape
- PMID: 37772967
- PMCID: PMC10827316
- DOI: 10.1093/plcell/koad253
The plant siRNA landscape
Abstract
Whereas micro (mi)RNAs are considered the clean, noble side of the small RNA world, small interfering (si)RNAs are often seen as a noisy set of molecules whose barbarian acronyms reflect a large diversity of often elusive origins and functions. Twenty-five years after their discovery in plants, however, new classes of siRNAs are still being identified, sometimes in discrete tissues or at particular developmental stages, making the plant siRNA world substantially more complex and subtle than originally anticipated. Focusing primarily on the model Arabidopsis, we review here the plant siRNA landscape, including transposable elements (TE)-derived siRNAs, a vast array of non-TE-derived endogenous siRNAs, as well as exogenous siRNAs produced in response to invading nucleic acids such as viruses or transgenes. We primarily emphasize the extraordinary sophistication and diversity of their biogenesis and, secondarily, the variety of their known or presumed functions, including via non-cell autonomous activities, in the sporophyte, gametophyte, and shortly after fertilization.
© The Author(s) 2023. Published by Oxford University Press on behalf of American Society of Plant Biologists.
Conflict of interest statement
Conflict of interest statement. None declared.
Figures
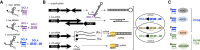
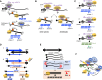
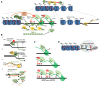
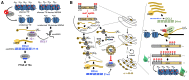
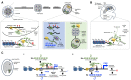
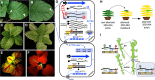
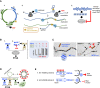
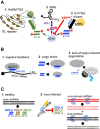
Similar articles
-
Genome-wide identification of genes regulated in trans by transposable element small interfering RNAs.RNA Biol. 2013 Aug;10(8):1379-95. doi: 10.4161/rna.25555. Epub 2013 Jul 2. RNA Biol. 2013. PMID: 23863322 Free PMC article.
-
Dual coding of siRNAs and miRNAs by plant transposable elements.RNA. 2008 May;14(5):814-21. doi: 10.1261/rna.916708. Epub 2008 Mar 26. RNA. 2008. PMID: 18367716 Free PMC article.
-
Genome-wide identification of endogenous RNA-directed DNA methylation loci associated with abundant 21-nucleotide siRNAs in Arabidopsis.Sci Rep. 2016 Oct 27;6:36247. doi: 10.1038/srep36247. Sci Rep. 2016. PMID: 27786269 Free PMC article.
-
The expanding world of small RNAs in plants.Nat Rev Mol Cell Biol. 2015 Dec;16(12):727-41. doi: 10.1038/nrm4085. Epub 2015 Nov 4. Nat Rev Mol Cell Biol. 2015. PMID: 26530390 Free PMC article. Review.
-
The diversity, biogenesis, and activities of endogenous silencing small RNAs in Arabidopsis.Annu Rev Plant Biol. 2014;65:473-503. doi: 10.1146/annurev-arplant-050213-035728. Epub 2014 Feb 26. Annu Rev Plant Biol. 2014. PMID: 24579988 Review.
Cited by
-
The Biosynthesis Process of Small RNA and Its Pivotal Roles in Plant Development.Int J Mol Sci. 2024 Jul 12;25(14):7680. doi: 10.3390/ijms25147680. Int J Mol Sci. 2024. PMID: 39062923 Free PMC article. Review.
-
Arabidopsis AGO1 N-terminal extension acts as an essential hub for PRMT5 interaction and post-translational modifications.Nucleic Acids Res. 2024 Aug 12;52(14):8466-8482. doi: 10.1093/nar/gkae387. Nucleic Acids Res. 2024. PMID: 38769059 Free PMC article.
-
An AGO10:miR165/6 module regulates meristem activity and xylem development in the Arabidopsis root.EMBO J. 2024 May;43(9):1843-1869. doi: 10.1038/s44318-024-00071-y. Epub 2024 Apr 2. EMBO J. 2024. PMID: 38565948 Free PMC article.
-
Functions and mechanisms of RNA tailing by nucleotidyl transferase proteins in plants.Front Plant Sci. 2024 Oct 15;15:1452347. doi: 10.3389/fpls.2024.1452347. eCollection 2024. Front Plant Sci. 2024. PMID: 39474218 Free PMC article. Review.
-
Targeted suppression of siRNA biogenesis in Arabidopsis pollen promotes triploid seed viability.Nat Commun. 2024 May 30;15(1):4612. doi: 10.1038/s41467-024-48950-6. Nat Commun. 2024. PMID: 38816386 Free PMC article.
References
Publication types
MeSH terms
Substances
LinkOut - more resources
Full Text Sources