Biomolecular condensates in plant RNA silencing: insights into formation, function, and stress responses
- PMID: 37772963
- PMCID: PMC10827315
- DOI: 10.1093/plcell/koad254
Biomolecular condensates in plant RNA silencing: insights into formation, function, and stress responses
Abstract
Biomolecular condensates are dynamic structures formed through diverse mechanisms, including liquid-liquid phase separation. These condensates have emerged as crucial regulators of cellular processes in eukaryotic cells, enabling the compartmentalization of specific biological reactions while allowing for dynamic exchange of molecules with the surrounding environment. RNA silencing, a conserved gene regulatory mechanism mediated by small RNAs (sRNAs), plays pivotal roles in various biological processes. Multiple types of biomolecular condensate, including dicing bodies, processing bodies, small interfering RNA bodies, and Cajal bodies, have been identified as key players in RNA silencing pathways. These biomolecular condensates provide spatial compartmentation for the biogenesis, loading, action, and turnover of small RNAs. Moreover, they actively respond to stresses, such as viral infections, and modulate RNA silencing activities during stress responses. This review summarizes recent advances in understanding of dicing bodies and other biomolecular condensates involved in RNA silencing. We explore their formation, roles in RNA silencing, and contributions to antiviral resistance responses. This comprehensive overview provides insights into the functional significance of biomolecular condensates in RNA silencing and expands our understanding of their roles in gene expression and stress responses in plants.
© American Society of Plant Biologists 2023. All rights reserved. For permissions, please e-mail: journals.permissions@oup.com.
Conflict of interest statement
Conflict of interest statement. The authors declare no competing interests, and we have included this information for all co-authors.
Figures
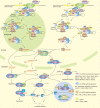
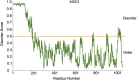
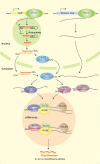
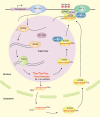
Similar articles
-
Cajal bodies: Evolutionarily conserved nuclear biomolecular condensates with properties unique to plants.Plant Cell. 2023 Sep 1;35(9):3214-3235. doi: 10.1093/plcell/koad140. Plant Cell. 2023. PMID: 37202374 Free PMC article. Review.
-
Stress-related biomolecular condensates in plants.Plant Cell. 2023 Sep 1;35(9):3187-3204. doi: 10.1093/plcell/koad127. Plant Cell. 2023. PMID: 37162152 Free PMC article.
-
Phase separation of chromatin and small RNA pathways in plants.Plant J. 2021 Dec;108(5):1256-1265. doi: 10.1111/tpj.15517. Epub 2021 Oct 9. Plant J. 2021. PMID: 34585805 Review.
-
Using quantitative reconstitution to investigate multicomponent condensates.RNA. 2022 Jan;28(1):27-35. doi: 10.1261/rna.079008.121. Epub 2021 Nov 12. RNA. 2022. PMID: 34772789 Free PMC article. Review.
-
Nuage condensates: accelerators or circuit breakers for sRNA silencing pathways?RNA. 2022 Jan;28(1):58-66. doi: 10.1261/rna.079003.121. Epub 2021 Nov 12. RNA. 2022. PMID: 34772788 Free PMC article. Review.
References
Publication types
MeSH terms
Substances
Grants and funding
LinkOut - more resources
Full Text Sources