The impact of Parkinson's disease-associated gut microbiota on the transcriptome in Drosophila
- PMID: 37754772
- PMCID: PMC10581176
- DOI: 10.1128/spectrum.00176-23
The impact of Parkinson's disease-associated gut microbiota on the transcriptome in Drosophila
Abstract
Parkinson's disease (PD) is a common neurodegenerative disease in middle-aged and elderly people, and many studies have confirmed that the disorder of gut microbiota is involved in the pathophysiological process of PD. However, the molecular mechanism of gut microbiota in regulating the pathogenesis of PD is still lacking. In this study, to investigate the impact of PD-associated gut microbiota on host transcriptome, we established various PD models with fecal microbiota transplantation (FMT) in the model organism Drosophila followed by integrative data analysis of microbiome and transcriptome. We first constructed rotenone-induced PD models in Drosophila followed by FMT in different groups. Microbial analysis by 16S rDNA sequencing showed that gut microbiota from PD Drosophila could affect bacterial structure of normal Drosophila, and gut microbiota from normal Drosophila could affect bacterial structure of PD Drosophila. Transcriptome analysis revealed that PD-associated gut microbiota influenced expression patterns of genes enriched in neuroactive ligand-receptor interaction, lysosome, and diverse metabolic pathways. Importantly, to verify our findings, we transplanted Drosophila with fecal samples from clinical PD patients. Compared to the control, Drosophila transplanted with fecal samples from PD patients had reduced microbiota Acetobacter and Lactobacillus, and differentially expressed genes enriched in diverse metabolic pathways. In summary, our results reveal the influence of PD-associated gut microbiota on host gene expression, and this study can help better understand the link between gut microbiota and PD pathogenesis through gut-brain axis. IMPORTANCE Gut microbiota plays important roles in regulating host gene expression and physiology through complex mechanisms. Recently, it has been suggested that disorder of gut microbiota is involved in the pathophysiological process of Parkinson's disease (PD). However, the molecular mechanism of gut microbiota in regulating the pathogenesis of PD is still lacking. In this study, to investigate the impact of PD-associated gut microbiota on host transcriptome, we established various PD models with fecal microbiota transplantation in the model organism Drosophila followed by integrative data analysis of microbiome and transcriptome. We also verified our findings by transplanting Drosophila with fecal samples from clinical PD patients. Our results demonstrated that PD-associated gut microbiota can induce differentially expressed genes enriched in diverse metabolic pathways. This study can help better understand the link between gut microbiota and PD pathogenesis through gut-brain axis.
Keywords: Drosophila melanogaster; Parkinson’s disease; fecal microbiota transplantation; gut microbiota; transcriptome.
Conflict of interest statement
The authors declare no conflict of interest.
Figures
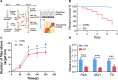
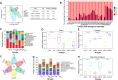
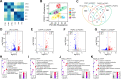
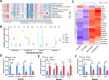
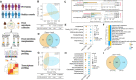
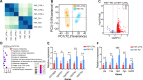
Similar articles
-
Fecal microbiota transplantation protects rotenone-induced Parkinson's disease mice via suppressing inflammation mediated by the lipopolysaccharide-TLR4 signaling pathway through the microbiota-gut-brain axis.Microbiome. 2021 Nov 17;9(1):226. doi: 10.1186/s40168-021-01107-9. Microbiome. 2021. PMID: 34784980 Free PMC article.
-
Neuroprotective effects of fecal microbiota transplantation on MPTP-induced Parkinson's disease mice: Gut microbiota, glial reaction and TLR4/TNF-α signaling pathway.Brain Behav Immun. 2018 May;70:48-60. doi: 10.1016/j.bbi.2018.02.005. Epub 2018 Feb 20. Brain Behav Immun. 2018. PMID: 29471030
-
Evaluation of fecal microbiota transplantation in Parkinson's disease patients with constipation.Microb Cell Fact. 2021 May 13;20(1):98. doi: 10.1186/s12934-021-01589-0. Microb Cell Fact. 2021. PMID: 33985520 Free PMC article.
-
[Advances in fecal microbiota transplantation for treatment of Parkinson's disease].Sheng Wu Gong Cheng Xue Bao. 2021 Nov 25;37(11):3812-3819. doi: 10.13345/j.cjb.200770. Sheng Wu Gong Cheng Xue Bao. 2021. PMID: 34841786 Review. Chinese.
-
Brain-gut-microbiota axis in Parkinson's disease: A historical review and future perspective.Brain Res Bull. 2022 Jun 1;183:84-93. doi: 10.1016/j.brainresbull.2022.02.015. Epub 2022 Mar 1. Brain Res Bull. 2022. PMID: 35245613 Review.
References
-
- Hill-Burns EM, Debelius JW, Morton JT, Wissemann WT, Lewis MR, Wallen ZD, Peddada SD, Factor SA, Molho E, Zabetian CP, Knight R, Payami H. 2017. Parkinson's disease and Parkinson's disease medications have distinct signatures of the gut microbiome. Mov Disord 32:739–749. doi:10.1002/mds.26942 - DOI - PMC - PubMed
-
- Cryan JF, O’Riordan KJ, Cowan CSM, Sandhu KV, Bastiaanssen TFS, Boehme M, Codagnone MG, Cussotto S, Fulling C, Golubeva AV, Guzzetta KE, Jaggar M, Long-Smith CM, Lyte JM, Martin JA, Molinero-Perez A, Moloney G, Morelli E, Morillas E, O’Connor R, Cruz-Pereira JS, Peterson VL, Rea K, Ritz NL, Sherwin E, Spichak S, Teichman EM, van de Wouw M, Ventura-Silva AP, Wallace-Fitzsimons SE, Hyland N, Clarke G, Dinan TG. 2019. The microbiota-gut-brain axis. Physiol Rev 99:1877–2013. doi:10.1152/physrev.00018.2018 - DOI - PubMed
-
- Sampson TR, Debelius JW, Thron T, Janssen S, Shastri GG, Ilhan ZE, Challis C, Schretter CE, Rocha S, Gradinaru V, Chesselet M-F, Keshavarzian A, Shannon KM, Krajmalnik-Brown R, Wittung-Stafshede P, Knight R, Mazmanian SK. 2016. Gut microbiota regulate motor deficits and neuroinflammation in a model of Parkinson's disease. Cell 167:1469–1480. doi:10.1016/j.cell.2016.11.018 - DOI - PMC - PubMed
LinkOut - more resources
Full Text Sources
Molecular Biology Databases