Vacuolar proteases and autophagy in phytopathogenic fungi: A review
- PMID: 37746183
- PMCID: PMC10512327
- DOI: 10.3389/ffunb.2022.948477
Vacuolar proteases and autophagy in phytopathogenic fungi: A review
Abstract
Autophagy (macroautophagy) is a survival and virulence mechanism of different eukaryotic pathogens. Autophagosomes sequester cytosolic material and organelles, then fuse with or enter into the vacuole or lysosome (the lytic compartment of most fungal/plant cells and many animal cells, respectively). Subsequent degradation of cargoes delivered to the vacuole via autophagy and endocytosis maintains cellular homeostasis and survival in conditions of stress, cellular differentiation, and development. PrA and PrB are vacuolar aspartyl and serine endoproteases, respectively, that participate in the autophagy of fungi and contribute to the pathogenicity of phytopathogens. Whereas the levels of vacuolar proteases are regulated by the expression of the genes encoding them (e.g., PEP4 for PrA and PRB1 for PrB), their activity is governed by endogenous inhibitors. The aim of the current contribution is to review the main characteristics, regulation, and role of vacuolar soluble endoproteases and Atg proteins in the process of autophagy and the pathogenesis of three fungal phytopathogens: Ustilago maydis, Magnaporthe oryzae, and Alternaria alternata. Aspartyl and serine proteases are known to participate in autophagy in these fungi by degrading autophagic bodies. However, the gene responsible for encoding the vacuolar serine protease of U. maydis has yet to be identified. Based on in silico analysis, this U. maydis gene is proposed to be orthologous to the Saccharomyces cerevisiae genes PRB1 and PBI2, known to encode the principal protease involved in the degradation of autophagic bodies and its inhibitor, respectively. In fungi that interact with plants, whether phytopathogenic or mycorrhizal, autophagy is a conserved cellular degradation process regulated through the TOR, PKA, and SNF1 pathways by ATG proteins and vacuolar proteases. Autophagy plays a preponderant role in the recycling of cell components as well as in the fungus-plant interaction.
Keywords: ATG8 and TOR; autophagic body degradation; autophagy; phytopathogenic fungus; vacuolar proteases PrA and PrB.
Copyright © 2022 Juárez-Montiel, Clark-Flores, Tesillo-Moreno, de la Vega-Camarillo, Andrade-Pavón, Hernández-García, Hernández-Rodríguez and Villa-Tanaca.
Conflict of interest statement
The authors declare that the research was conducted in the absence of any commercial or financial relationships that could be construed as a potential conflict of interest.
Figures
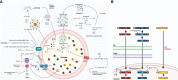
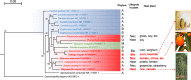
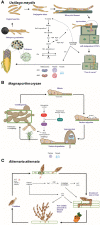
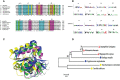
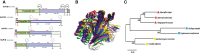
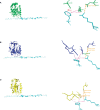
Similar articles
-
Vac8 Controls Vacuolar Membrane Dynamics during Different Autophagy Pathways in Saccharomyces cerevisiae.Cells. 2019 Jun 30;8(7):661. doi: 10.3390/cells8070661. Cells. 2019. PMID: 31262095 Free PMC article.
-
A Cinderella story: how the vacuolar proteases Pep4 and Prb1 do more than cleaning up the cell's mass degradation processes.Microb Cell. 2018 Aug 18;5(10):438-443. doi: 10.15698/mic2018.10.650. Microb Cell. 2018. PMID: 30386788 Free PMC article. Review.
-
Aaprb1, a subtilsin-like protease, required for autophagy and virulence of the tangerine pathotype of Alternaria alternata.Microbiol Res. 2020 Nov;240:126537. doi: 10.1016/j.micres.2020.126537. Epub 2020 Jun 29. Microbiol Res. 2020. PMID: 32739584
-
Vacuolar proteases from Candida glabrata: Acid aspartic protease PrA, neutral serine protease PrB and serine carboxypeptidase CpY. The nitrogen source influences their level of expression.Rev Iberoam Micol. 2016 Jan-Mar;33(1):26-33. doi: 10.1016/j.riam.2014.10.005. Epub 2015 Jul 16. Rev Iberoam Micol. 2016. PMID: 26422323
-
Vacuolar Processing Enzymes in Plant Programmed Cell Death and Autophagy.Int J Mol Sci. 2023 Jan 7;24(2):1198. doi: 10.3390/ijms24021198. Int J Mol Sci. 2023. PMID: 36674706 Free PMC article. Review.
References
Publication types
LinkOut - more resources
Full Text Sources
Molecular Biology Databases
Research Materials