The oral microbiome: diversity, biogeography and human health
- PMID: 37700024
- PMCID: PMC11084736
- DOI: 10.1038/s41579-023-00963-6
The oral microbiome: diversity, biogeography and human health
Abstract
The human oral microbiota is highly diverse and has a complex ecology, comprising bacteria, microeukaryotes, archaea and viruses. These communities have elaborate and highly structured biogeography that shapes metabolic exchange on a local scale and results from the diverse microenvironments present in the oral cavity. The oral microbiota also interfaces with the immune system of the human host and has an important role in not only the health of the oral cavity but also systemic health. In this Review, we highlight recent advances including novel insights into the biogeography of several oral niches at the species level, as well as the ecological role of candidate phyla radiation bacteria and non-bacterial members of the oral microbiome. In addition, we summarize the relationship between the oral microbiota and the pathology of oral diseases and systemic diseases. Together, these advances move the field towards a more holistic understanding of the oral microbiota and its role in health, which in turn opens the door to the study of novel preventive and therapeutic strategies.
© 2023. Springer Nature Limited.
Conflict of interest statement
Competing interests
The authors declare no competing interests.
Figures
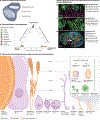
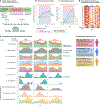
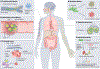
Similar articles
-
Ecology of the Oral Microbiome: Beyond Bacteria.Trends Microbiol. 2017 May;25(5):362-374. doi: 10.1016/j.tim.2016.12.012. Epub 2017 Jan 11. Trends Microbiol. 2017. PMID: 28089325 Free PMC article. Review.
-
Human oral microbiota and its modulation for oral health.Biomed Pharmacother. 2018 Mar;99:883-893. doi: 10.1016/j.biopha.2018.01.146. Epub 2018 Feb 20. Biomed Pharmacother. 2018. PMID: 29710488 Review.
-
The oral microbiome in health and disease.Pharmacol Res. 2013 Mar;69(1):137-43. doi: 10.1016/j.phrs.2012.11.006. Epub 2012 Nov 28. Pharmacol Res. 2013. PMID: 23201354 Review.
-
Interactions between systemic diseases and oral microbiota shifts in the aging community: A narrative review.J Basic Microbiol. 2023 Aug;63(8):831-854. doi: 10.1002/jobm.202300141. Epub 2023 May 12. J Basic Microbiol. 2023. PMID: 37173818 Review.
-
The oral microbiome diversity and its relation to human diseases.Folia Microbiol (Praha). 2015 Jan;60(1):69-80. doi: 10.1007/s12223-014-0342-2. Epub 2014 Aug 23. Folia Microbiol (Praha). 2015. PMID: 25147055 Review.
Cited by
-
Effect of Dietary Composite Probiotic Supplementation on the Microbiota of Different Oral Sites in Cats.Vet Sci. 2024 Aug 4;11(8):351. doi: 10.3390/vetsci11080351. Vet Sci. 2024. PMID: 39195805 Free PMC article.
-
Ethanol extract from Astilbe chinensis inflorescence suppresses inflammation in macrophages and growth of oral pathogenic bacteria.PLoS One. 2024 Jul 3;19(7):e0306543. doi: 10.1371/journal.pone.0306543. eCollection 2024. PLoS One. 2024. PMID: 38959234 Free PMC article.
-
Effect of chronic alcohol consumption on oral microbiota in rats with periodontitis.PeerJ. 2024 Aug 12;12:e17795. doi: 10.7717/peerj.17795. eCollection 2024. PeerJ. 2024. PMID: 39148678 Free PMC article.
-
Insights into the enigma of oral streptococci in carcinogenesis.Microbiol Mol Biol Rev. 2024 Jun 27;88(2):e0009523. doi: 10.1128/mmbr.00095-23. Epub 2024 Mar 20. Microbiol Mol Biol Rev. 2024. PMID: 38506551 Review.
-
The Baker Lab at the OHSU School of Dentistry: leveraging bioinformatics and molecular biology to discover how the bacteria that live in our mouth impact human health and disease.OHSU Sch Dent Anthol. 2023 Winter;1(1):3-11. doi: 10.6083/bpxhc42395. OHSU Sch Dent Anthol. 2023. PMID: 38784447 Free PMC article.
References
-
- Dobell C Antony van Leeuwenhoek and His ‘Little Animals’: Being Some Account of the Father of Protozoology and Bacteriology and His Multifarious Discoveries in these Disciplines (Harcourt, Brace and Company, 1932).
Publication types
MeSH terms
Grants and funding
LinkOut - more resources
Full Text Sources