Ryanodine Receptor Staining Identifies Viable Cardiomyocytes in Human and Rabbit Cardiac Tissue Slices
- PMID: 37686327
- PMCID: PMC10488113
- DOI: 10.3390/ijms241713514
Ryanodine Receptor Staining Identifies Viable Cardiomyocytes in Human and Rabbit Cardiac Tissue Slices
Abstract
In terms of preserving multicellularity and myocardial function in vitro, the cultivation of beating myocardial slices is an emerging technique in basic and translational cardiac research. It can be used, for example, for drug screening or to study pathomechanisms. Here, we describe staining for viable cardiomyocytes based on the immunofluorescence of ryanodine receptors (RyRs) in human and rabbit myocardial slices. Biomimetic chambers were used for culture and measurements of contractile force. Fixable fluorophore-conjugated dextran, entering cells with a permeable membrane, was used for death staining. RyRs, nuclei and the extracellular matrix, including the t-system, were additionally stained and analyzed by confocal microscopy and image processing. We found the mutual exclusion of the RyR and dextran signals in cultivated slices. T-System density and nucleus size were reduced in RyR-negative/dextran-positive myocytes. The fraction of RyR-positive myocytes and pixels correlated with the contractile force. In RyR-positive/dextran-positive myocytes, we found irregular RyR clusters and SERCA distribution patterns, confirmed by an altered power spectrum. We conclude that RyR immunofluorescence indicates viable cardiomyocytes in vibratome-cut myocardial slices, facilitating the detection and differential structural analysis of living vs. dead or dying myocytes. We suggest the loss of sarcoplasmic reticulum integrity as an early event during cardiomyocyte death.
Keywords: cardiac tissue slices; death staining; ryanodine receptor; sarcoplasmic reticulum; viability assay.
Conflict of interest statement
T.S. is a shareholder of InVitroSys GmbH.
Figures
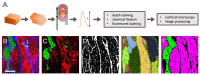
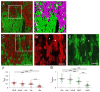
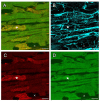
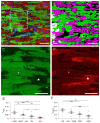
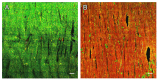
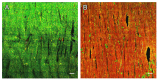
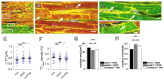
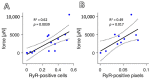
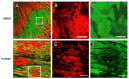
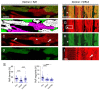
Similar articles
-
Oxidation of ryanodine receptor after ischemia-reperfusion increases propensity of Ca2+ waves during β-adrenergic receptor stimulation.Am J Physiol Heart Circ Physiol. 2018 Oct 1;315(4):H1032-H1040. doi: 10.1152/ajpheart.00334.2018. Epub 2018 Jul 20. Am J Physiol Heart Circ Physiol. 2018. PMID: 30028204 Free PMC article.
-
Redox modification of ryanodine receptors by mitochondria-derived reactive oxygen species contributes to aberrant Ca2+ handling in ageing rabbit hearts.J Physiol. 2013 Dec 1;591(23):5895-911. doi: 10.1113/jphysiol.2013.260521. Epub 2013 Sep 16. J Physiol. 2013. PMID: 24042501 Free PMC article.
-
A novel mechanism of tandem activation of ryanodine receptors by cytosolic and SR luminal Ca2+ during excitation-contraction coupling in atrial myocytes.J Physiol. 2017 Jun 15;595(12):3835-3845. doi: 10.1113/JP273611. Epub 2017 Feb 1. J Physiol. 2017. PMID: 28028837 Free PMC article.
-
Ca2+ stores regulate ryanodine receptor Ca2+ release channels via luminal and cytosolic Ca2+ sites.Clin Exp Pharmacol Physiol. 2007 Sep;34(9):889-96. doi: 10.1111/j.1440-1681.2007.04708.x. Clin Exp Pharmacol Physiol. 2007. PMID: 17645636 Review.
-
Cardiac BIN1 (cBIN1) is a regulator of cardiac contractile function and an emerging biomarker of heart muscle health.Sci China Life Sci. 2017 Mar;60(3):257-263. doi: 10.1007/s11427-016-0249-x. Epub 2016 Nov 23. Sci China Life Sci. 2017. PMID: 27888388 Review.
Cited by
-
Effects of electro-mechanical uncouplers, hormonal stimulation and pacing rate on the stability and function of cultured rabbit myocardial slices.Front Bioeng Biotechnol. 2024 Apr 5;12:1363538. doi: 10.3389/fbioe.2024.1363538. eCollection 2024. Front Bioeng Biotechnol. 2024. PMID: 38646013 Free PMC article.
References
-
- Fischer C., Milting H., Fein E., Reiser E., Lu K., Seidel T., Schinner C., Schwarzmayr T., Schramm R., Tomasi R., et al. Long-term functional and structural preservation of precision-cut human myocardium under continuous electromechanical stimulation in vitro. Nat. Commun. 2019;10:117. doi: 10.1038/s41467-018-08003-1. - DOI - PMC - PubMed
-
- Abu-Khousa M., Fiegle D.J., Sommer S.T., Minabari G., Milting H., Heim C., Weyand M., Tomasi R., Dendorfer A., Volk T., et al. The Degree of t-System Remodeling Predicts Negative Force-Frequency Relationship and Prolonged Relaxation Time in Failing Human Myocardium. Front. Physiol. 2020;11:182. doi: 10.3389/fphys.2020.00182. - DOI - PMC - PubMed
MeSH terms
Substances
Grants and funding
LinkOut - more resources
Full Text Sources