Plk2 promotes synaptic destabilization through disruption of N-cadherin adhesion complexes during homeostatic adaptation to hyperexcitation
- PMID: 37654026
- PMCID: PMC10592368
- DOI: 10.1111/jnc.15948
Plk2 promotes synaptic destabilization through disruption of N-cadherin adhesion complexes during homeostatic adaptation to hyperexcitation
Abstract
Synaptogenesis in the brain is highly organized and orchestrated by synaptic cellular adhesion molecules (CAMs) such as N-cadherin and amyloid precursor protein (APP) that contribute to the stabilization and structure of synapses. Although N-cadherin plays an integral role in synapse formation and synaptic plasticity, its function in synapse dismantling is not as well understood. Synapse weakening and loss are prominent features of neurodegenerative diseases, and can also be observed during homeostatic compensation to neuronal hyperexcitation. Previously, we have shown that during homeostatic synaptic plasticity, APP is a target for cleavage triggered by phosphorylation by Polo-like kinase 2 (Plk2). Here, we found that Plk2 directly phosphorylates N-cadherin, and during neuronal hyperexcitation Plk2 promotes N-cadherin proteolytic processing, degradation, and disruption of complexes with APP. We further examined the molecular mechanisms underlying N-cadherin degradation. Loss of N-cadherin adhesive function destabilizes excitatory synapses and promotes their structural dismantling as a prerequisite to eventual synapse elimination. This pathway, which may normally help to homeostatically restrain excitability, could also shed light on the dysregulated synapse loss that occurs in cognitive disorders.
Keywords: APP; N-cadherin; Plk2; homeostatic synaptic plasticity; hyperexcitation; synapse loss.
© 2023 The Authors. Journal of Neurochemistry published by John Wiley & Sons Ltd on behalf of International Society for Neurochemistry.
Conflict of interest statement
CONFLICT OF INTEREST STATEMENT
The authors have no conflicts of interest to declare.
Figures
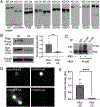
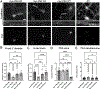
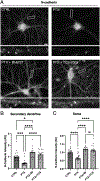
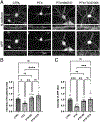
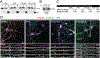
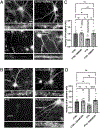
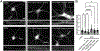
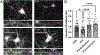
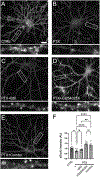
Similar articles
-
Polo-like kinase 2 phosphorylation of amyloid precursor protein regulates activity-dependent amyloidogenic processing.Neuropharmacology. 2017 May 1;117:387-400. doi: 10.1016/j.neuropharm.2017.02.027. Epub 2017 Mar 1. Neuropharmacology. 2017. PMID: 28257888 Free PMC article.
-
Plk2 attachment to NSF induces homeostatic removal of GluA2 during chronic overexcitation.Nat Neurosci. 2010 Oct;13(10):1199-207. doi: 10.1038/nn.2624. Epub 2010 Aug 29. Nat Neurosci. 2010. PMID: 20802490 Free PMC article.
-
Inhibition of Polo-like kinase 2 ameliorates pathogenesis in Alzheimer's disease model mice.PLoS One. 2019 Jul 15;14(7):e0219691. doi: 10.1371/journal.pone.0219691. eCollection 2019. PLoS One. 2019. PMID: 31306446 Free PMC article.
-
Regulation of neuronal PKA signaling through AKAP targeting dynamics.Eur J Cell Biol. 2006 Jul;85(7):627-33. doi: 10.1016/j.ejcb.2006.01.010. Epub 2006 Feb 28. Eur J Cell Biol. 2006. PMID: 16504338 Review.
-
Cell adhesion and homeostatic synaptic plasticity.Neuropharmacology. 2014 Mar;78:23-30. doi: 10.1016/j.neuropharm.2013.03.015. Epub 2013 Mar 28. Neuropharmacology. 2014. PMID: 23542441 Review.
References
-
- Amini M, Ma C-L, Farazifard R, Zhu G, Zhang Y, Vanderluit J, Zoltewicz JS, Hage F, Savitt JM, Lagace DC, Slack RS, Beique J-C, Baudry M, Greer PA, Bergeron R, & Park DS (2013). Cellular/Molecular Conditional Disruption of Calpain in the CNS Alters Dendrite Morphology, Impairs LTP, and Promotes Neuronal Survival following Injury. 10.1523/JNEUROSCI.4247-12.2013 - DOI - PMC - PubMed
-
- Ando K, Uemura K, Kuzuya A, Maesako M, Asada-Utsugi M, Kubota M, Aoyagi N, Yoshioka K, Okawa K, Inoue H, Kawamata J, Shimohama S, Arai T, Takahashi R, & Kinoshita A (2011). N-cadherin regulates p38 MAPK signaling via association with JNK-associated leucine zipper protein: implications for neurodegeneration in Alzheimer disease. The Journal of Biological Chemistry, 286(9), 7619–7628. 10.1074/jbc.M110.158477 - DOI - PMC - PubMed
-
- Asada-Utsugi M, Uemura K, Kubota M, Noda Y, Tashiro Y, Uemura TM, Yamakado H, Urushitani M, Takahashi R, Hattori S, Miyakawa T, Ageta-Ishihara N, Kobayashi K, Kinoshita M, & Kinoshita A (2021). Mice with cleavage-resistant N-cadherin exhibit synapse anomaly in the hippocampus and outperformance in spatial learning tasks. Molecular Brain, 14(1), 1–16. 10.1186/S13041-021-00738-1/FIGURES/8 - DOI - PMC - PubMed
Publication types
MeSH terms
Substances
Grants and funding
LinkOut - more resources
Full Text Sources
Research Materials