Endothelial RIPK1 protects artery bypass graft against arteriosclerosis by regulating SMC growth
- PMID: 37647392
- PMCID: PMC10468134
- DOI: 10.1126/sciadv.adh8939
Endothelial RIPK1 protects artery bypass graft against arteriosclerosis by regulating SMC growth
Abstract
RIPK1 is crucial in the inflammatory response. The process of vascular graft remodeling is also involved in endothelial inflammation, which can influence the behavior of smooth muscle cells. However, the role of endothelial RIPK1 in arterial bypass grafts remains unknown. Here, we established an arterial isograft mouse model in wild-type and endothelial RIPK1 conditional knockout mice. Progressive vascular remodeling and neointima formation occurred in the graft artery, showing SMC accumulation together with endothelial inflammatory adhesion molecule and cytokine expression. Endothelial RIPK1 knockout exacerbated graft stenosis by increasing secretion of N-Shh. Mechanistically, RIPK1 directly phosphorylated EEF1AKMT3 at Ser26, inhibiting its methyltransferase activity and global protein synthesis, which further attenuated N-Shh translation and secretion. Consistently, treatment with the Hedgehog pathway inhibitor GDC0449 markedly alleviated RIPK1 knockout-induced graft stenosis. Our results demonstrated that endothelial RIPK1 played a protective role in arterial bypass graft vascular remodeling, highlighting that targeting Hedgehog pathway may be an attractive strategy for graft failure in the future.
Figures
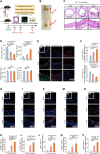
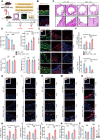
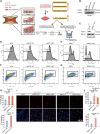
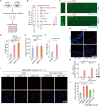
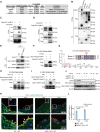
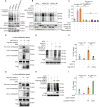
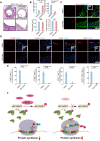
Similar articles
-
SOCS1 prevents graft arteriosclerosis by preserving endothelial cell function.J Am Coll Cardiol. 2014 Jan 7-14;63(1):21-9. doi: 10.1016/j.jacc.2013.08.694. Epub 2013 Aug 28. J Am Coll Cardiol. 2014. PMID: 23994402 Free PMC article.
-
Inhibition of Smooth Muscle β-Catenin Hinders Neointima Formation After Vascular Injury.Arterioscler Thromb Vasc Biol. 2017 May;37(5):879-888. doi: 10.1161/ATVBAHA.116.308643. Epub 2017 Mar 16. Arterioscler Thromb Vasc Biol. 2017. PMID: 28302627 Free PMC article.
-
Aldosterone promotes vascular remodeling by direct effects on smooth muscle cell mineralocorticoid receptors.Arterioscler Thromb Vasc Biol. 2014 Feb;34(2):355-64. doi: 10.1161/ATVBAHA.113.302854. Epub 2013 Dec 5. Arterioscler Thromb Vasc Biol. 2014. PMID: 24311380 Free PMC article.
-
Mature Vascular Smooth Muscle Cells, but Not Endothelial Cells, Serve as the Major Cellular Source of Intimal Hyperplasia in Vein Grafts.Arterioscler Thromb Vasc Biol. 2020 Aug;40(8):1870-1890. doi: 10.1161/ATVBAHA.120.314465. Epub 2020 Jun 4. Arterioscler Thromb Vasc Biol. 2020. PMID: 32493169 Free PMC article.
-
Mouse models of arteriosclerosis: from arterial injuries to vascular grafts.Am J Pathol. 2004 Jul;165(1):1-10. doi: 10.1016/S0002-9440(10)63270-1. Am J Pathol. 2004. PMID: 15215157 Free PMC article. Review.
Cited by
-
NQO1 promotes osteogenesis and suppresses angiogenesis in DPSCs via MAPK pathway modulation.Stem Cell Res Ther. 2024 Sep 16;15(1):306. doi: 10.1186/s13287-024-03929-4. Stem Cell Res Ther. 2024. PMID: 39285500 Free PMC article.
References
-
- D. Thuijs, A. Kappetein, P. Serruys, F. Mohr, M. Morice, M. Mack, D. Holmes, N. Curzen, P. Davierwala, T. Noack, M. Milojevic, K. Dawkins, B. da Costa, P. Jüni, S. Head, Percutaneous coronary intervention versus coronary artery bypass grafting in patients with three-vessel or left main coronary artery disease: 10-year follow-up of the multicentre randomised controlled SYNTAX trial. Lancet 394, 1325–1334 (2019). - PubMed
-
- M. Gaudino, U. Benedetto, S. Fremes, G. Biondi-Zoccai, A. Sedrakyan, J. Puskas, G. Angelini, B. Buxton, G. Frati, D. Hare, P. Hayward, G. Nasso, N. Moat, M. Peric, K. Yoo, G. Speziale, L. Girardi, D. Taggart, Radial-artery or saphenous-vein grafts in coronary-artery bypass surgery. N. Engl. J. Med. 378, 2069–2077 (2018). - PubMed
-
- M. Gaudino, U. Benedetto, S. Fremes, K. Ballman, G. Biondi-Zoccai, A. Sedrakyan, G. Nasso, J. Raman, B. Buxton, P. Hayward, N. Moat, P. Collins, C. Webb, M. Peric, I. Petrovic, K. Yoo, I. Hameed, A. Di Franco, M. Moscarelli, G. Speziale, J. Puskas, L. Girardi, D. Hare, D. Taggart, Association of radial artery graft vs saphenous vein graft with long-term cardiovascular outcomes among patients undergoing coronary artery bypass grafting: A systematic review and meta-analysis. JAMA 324, 179–187 (2020). - PMC - PubMed
-
- R. Mitchell, P. Libby, Vascular remodeling in transplant vasculopathy. Circ. Res. 100, 967–978 (2007). - PubMed
-
- J. Pober, W. Sessa, Evolving functions of endothelial cells in inflammation. Nat. Rev. Immunol. 7, 803–815 (2007). - PubMed
MeSH terms
Substances
LinkOut - more resources
Full Text Sources
Miscellaneous