Age-related decline in cognitive flexibility is associated with the levels of hippocampal neurogenesis
- PMID: 37645372
- PMCID: PMC10461065
- DOI: 10.3389/fnins.2023.1232670
Age-related decline in cognitive flexibility is associated with the levels of hippocampal neurogenesis
Abstract
Aging is associated with impairments in learning, memory, and cognitive flexibility, as well as a gradual decline in hippocampal neurogenesis. We investigated the performance of 6-and 14-month-old mice (considered mature adult and late middle age, respectively) in learning and memory tasks based on the Morris water maze (MWM) and determined their levels of preceding and current neurogenesis. While both age groups successfully performed in the spatial version of MWM (sMWM), the older mice were less efficient compared to the younger mice when presented with modified versions of the MWM that required a reassessment of the previously acquired experience. This was detected in the reversal version of MWM (rMWM) and was particularly evident in the context discrimination MWM (cdMWM), a novel task that required integrating various distal cues, local cues, and altered contexts and adjusting previously used search strategies. Older mice were impaired in several metrics that characterize rMWM and cdMWM, however, they showed improvement and narrowed the performance gap with the younger mice after additional training. Furthermore, we analyzed the adult-born mature and immature neurons in the hippocampal dentate gyrus and found a significant correlation between neurogenesis levels in individual mice and their performance in the tasks demanding cognitive flexibility. These results provide a detailed description of the age-related changes in learning and memory and underscore the importance of hippocampal neurogenesis in supporting cognitive flexibility.
Keywords: adult-born neurons; aging; cognitive flexibility; hippocampal neurogenesis; neuronal maturation; search strategies; spatial learning.
Copyright © 2023 Amelchenko, Bezriadnov, Chekhov, Anokhin, Lazutkin and Enikolopov.
Conflict of interest statement
The authors declare that the research was conducted in the absence of any commercial or financial relationships that could be construed as a potential conflict of interest.
Figures
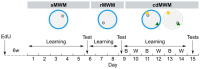
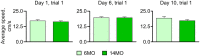
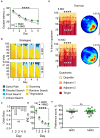
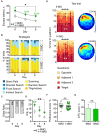
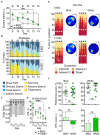
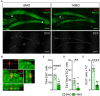
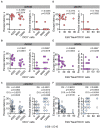
Similar articles
-
Cognitive Flexibility Is Selectively Impaired by Radiation and Is Associated with Differential Recruitment of Adult-Born Neurons.J Neurosci. 2023 Aug 23;43(34):6061-6083. doi: 10.1523/JNEUROSCI.0161-22.2023. Epub 2023 Aug 2. J Neurosci. 2023. PMID: 37532464 Free PMC article.
-
Early Stroke Induces Long-Term Impairment of Adult Neurogenesis Accompanied by Hippocampal-Mediated Cognitive Decline.Cells. 2019 Dec 17;8(12):1654. doi: 10.3390/cells8121654. Cells. 2019. PMID: 31861141 Free PMC article.
-
Spatial Navigation (Water Maze) Tasks.In: Buccafusco JJ, editor. Methods of Behavior Analysis in Neuroscience. 2nd edition. Boca Raton (FL): CRC Press/Taylor & Francis; 2009. Chapter 13. In: Buccafusco JJ, editor. Methods of Behavior Analysis in Neuroscience. 2nd edition. Boca Raton (FL): CRC Press/Taylor & Francis; 2009. Chapter 13. PMID: 21204326 Free Books & Documents. Review.
-
Spatial learning and neurogenesis: Effects of cessation of wheel running and survival of novel neurons by engagement in cognitive tasks.Hippocampus. 2016 Jun;26(6):794-803. doi: 10.1002/hipo.22560. Epub 2016 Jan 18. Hippocampus. 2016. PMID: 26669934
-
Morris Water Maze and Contextual Fear Conditioning Tasks to Evaluate Cognitive Functions Associated With Adult Hippocampal Neurogenesis.Front Neurosci. 2022 Jan 3;15:782947. doi: 10.3389/fnins.2021.782947. eCollection 2021. Front Neurosci. 2022. PMID: 35046769 Free PMC article. Review.
Cited by
-
The Pgb1 locus controls glycogen aggregation in astrocytes of the aged hippocampus without impacting cognitive function.bioRxiv [Preprint]. 2024 Jul 8:2023.11.22.567373. doi: 10.1101/2023.11.22.567373. bioRxiv. 2024. PMID: 38045339 Free PMC article. Preprint.
References
-
- Amelchenko E., Bezrriadnov D. V., Chekhov O. A., Ivanova A., Kedrov A. V., Anokhin K. V., et al. . (2023). Cognitive flexibility is selectively impaired by radiation and is associated with differential recruitment of adult-born neurons. J. Neurosci. Press. doi: 10.1523/JNEUROSCI.0161-22.2023 - DOI - PMC - PubMed
Grants and funding
LinkOut - more resources
Full Text Sources
Miscellaneous