In vivo recording of suprachiasmatic nucleus dynamics reveals a dominant role of arginine vasopressin neurons in circadian pacesetting
- PMID: 37643163
- PMCID: PMC10465001
- DOI: 10.1371/journal.pbio.3002281
In vivo recording of suprachiasmatic nucleus dynamics reveals a dominant role of arginine vasopressin neurons in circadian pacesetting
Abstract
The central circadian clock of the suprachiasmatic nucleus (SCN) is a network consisting of various types of neurons and glial cells. Individual cells have the autonomous molecular machinery of a cellular clock, but their intrinsic periods vary considerably. Here, we show that arginine vasopressin (AVP) neurons set the ensemble period of the SCN network in vivo to control the circadian behavior rhythm. Artificial lengthening of cellular periods by deleting casein kinase 1 delta (CK1δ) in the whole SCN lengthened the free-running period of behavior rhythm to an extent similar to CK1δ deletion specific to AVP neurons. However, in SCN slices, PER2::LUC reporter rhythms of these mice only partially and transiently recapitulated the period lengthening, showing a dissociation between the SCN shell and core with a period instability in the shell. In contrast, in vivo calcium rhythms of both AVP and vasoactive intestinal peptide (VIP) neurons in the SCN of freely moving mice demonstrated stably lengthened periods similar to the behavioral rhythm upon AVP neuron-specific CK1δ deletion, without changing the phase relationships between each other. Furthermore, optogenetic activation of AVP neurons acutely induced calcium increase in VIP neurons in vivo. These results indicate that AVP neurons regulate other SCN neurons, such as VIP neurons, in vivo and thus act as a primary determinant of the SCN ensemble period.
Copyright: © 2023 Tsuno et al. This is an open access article distributed under the terms of the Creative Commons Attribution License, which permits unrestricted use, distribution, and reproduction in any medium, provided the original author and source are credited.
Conflict of interest statement
The authors have declared that no competing interests exist.
Figures
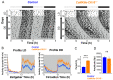
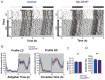
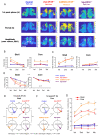
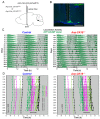
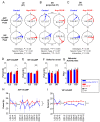
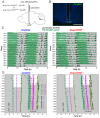
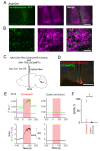
Similar articles
-
The transcription factor VAX1 in VIP neurons of the suprachiasmatic nucleus impacts circadian rhythm generation, depressive-like behavior, and the reproductive axis in a sex-specific manner in mice.Front Endocrinol (Lausanne). 2023 Dec 22;14:1269672. doi: 10.3389/fendo.2023.1269672. eCollection 2023. Front Endocrinol (Lausanne). 2023. PMID: 38205198 Free PMC article.
-
Manipulating the Cellular Circadian Period of Arginine Vasopressin Neurons Alters the Behavioral Circadian Period.Curr Biol. 2016 Sep 26;26(18):2535-2542. doi: 10.1016/j.cub.2016.07.022. Epub 2016 Aug 25. Curr Biol. 2016. PMID: 27568590
-
Loss of arginine vasopressin- and vasoactive intestinal polypeptide-containing neurons and glial cells in the suprachiasmatic nucleus of individuals with type 2 diabetes.Diabetologia. 2019 Nov;62(11):2088-2093. doi: 10.1007/s00125-019-4953-7. Epub 2019 Jul 20. Diabetologia. 2019. PMID: 31327049 Free PMC article.
-
Vasopressin in circadian function of SCN.J Biosci. 2020;45:140. J Biosci. 2020. PMID: 33361631 Review.
-
[Mechanisms of structural plasticity associated with photic synchronization of the circadian clock within the suprachiasmatic nucleus].J Soc Biol. 2009;203(1):49-63. doi: 10.1051/jbio:2009004. Epub 2009 Apr 10. J Soc Biol. 2009. PMID: 19358811 Review. French.
Cited by
-
The transcription factor VAX1 in VIP neurons of the suprachiasmatic nucleus impacts circadian rhythm generation, depressive-like behavior, and the reproductive axis in a sex-specific manner in mice.Front Endocrinol (Lausanne). 2023 Dec 22;14:1269672. doi: 10.3389/fendo.2023.1269672. eCollection 2023. Front Endocrinol (Lausanne). 2023. PMID: 38205198 Free PMC article.
-
In vivo recording of the circadian calcium rhythm in Prokineticin 2 neurons of the suprachiasmatic nucleus.Sci Rep. 2023 Oct 9;13(1):16974. doi: 10.1038/s41598-023-44282-5. Sci Rep. 2023. PMID: 37813987 Free PMC article.
-
Circadian rhythm mechanism in the suprachiasmatic nucleus and its relation to the olfactory system.Front Neural Circuits. 2024 Mar 25;18:1385908. doi: 10.3389/fncir.2024.1385908. eCollection 2024. Front Neural Circuits. 2024. PMID: 38590628 Free PMC article. Review.
References
Publication types
MeSH terms
Substances
Grants and funding
LinkOut - more resources
Full Text Sources
Molecular Biology Databases
Research Materials
Miscellaneous