This is a preprint.
Curved crease origami and topological singularities at a cellular scale enable hyper-extensibility of Lacrymaria olor
- PMID: 37577489
- PMCID: PMC10418517
- DOI: 10.1101/2023.08.04.551915
Curved crease origami and topological singularities at a cellular scale enable hyper-extensibility of Lacrymaria olor
Update in
-
Curved crease origami and topological singularities enable hyperextensibility of L. olor.Science. 2024 Jun 7;384(6700):eadk5511. doi: 10.1126/science.adk5511. Epub 2024 Jun 7. Science. 2024. PMID: 38843314
Abstract
Eukaryotic cells undergo dramatic morphological changes during cell division, phagocytosis and motility. Fundamental limits of cellular morphodynamics such as how fast or how much cellular shapes can change without harm to a living cell remain poorly understood. Here we describe hyper-extensibility in the single-celled protist Lacrymaria olor, a 40 μm cell which is capable of reversible and repeatable extensions (neck-like protrusions) up to 1500 μm in 30 seconds. We discover that a unique and intricate organization of cortical cytoskeleton and membrane enables these hyper-extensions that can be described as the first cellular scale curved crease origami. Furthermore, we show how these topological singularities including d-cones and twisted domain walls provide a geometrical control mechanism for the deployment of membrane and microtubule sheets as they repeatably spool thousands of time from the cell body. We lastly build physical origami models to understand how these topological singularities provide a mechanism for the cell to control the hyper-extensile deployable structure. This new geometrical motif where a cell employs curved crease origami to perform a physiological function has wide ranging implications in understanding cellular morphodynamics and direct applications in deployable micro-robotics.
Keywords: Active filaments; Biophysics; Cell-behavior; Non-linear dynamics; Protists; curved crease origami.
Figures
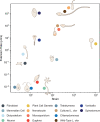
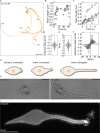
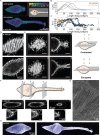
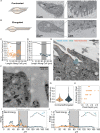
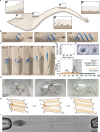
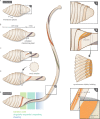
Similar articles
-
Curved crease origami and topological singularities enable hyperextensibility of L. olor.Science. 2024 Jun 7;384(6700):eadk5511. doi: 10.1126/science.adk5511. Epub 2024 Jun 7. Science. 2024. PMID: 38843314
-
Coupled Active Systems Encode an Emergent Hunting Behavior in the Unicellular Predator Lacrymaria olor.Curr Biol. 2019 Nov 18;29(22):3838-3850.e3. doi: 10.1016/j.cub.2019.09.034. Epub 2019 Oct 31. Curr Biol. 2019. PMID: 31679941 Free PMC article.
-
Dynamic shape-shifting of the single-celled eukaryotic predator Lacrymaria via unconventional cytoskeletal components.Curr Biol. 2024 Nov 4;34(21):4869-4883.e6. doi: 10.1016/j.cub.2024.09.003. Epub 2024 Sep 30. Curr Biol. 2024. PMID: 39353425
-
Architected Origami Materials: How Folding Creates Sophisticated Mechanical Properties.Adv Mater. 2019 Feb;31(5):e1805282. doi: 10.1002/adma.201805282. Epub 2018 Dec 5. Adv Mater. 2019. PMID: 30516852 Review.
-
Advancing Biophysics Using DNA Origami.Annu Rev Biophys. 2021 May 6;50:469-492. doi: 10.1146/annurev-biophys-110520-125739. Epub 2021 Mar 1. Annu Rev Biophys. 2021. PMID: 33646812 Review.
References
-
- Remedios C. G. D. et al. Actin binding proteins: Regulation of cytoskeletal microfilaments. Physiological Reviews 83, 433–473 (2003). - PubMed
-
- Yap B. & Kamm R. D. Cytoskeletal remodeling and cellular activation during deformation of neutrophils into narrow channels. Journal of Applied Physiology 99, 2323–2330 (2005). - PubMed
Publication types
Associated data
Grants and funding
LinkOut - more resources
Full Text Sources