Cholesterol-Depletion-Induced Membrane Repair Carries a Raft Conformer of P-Glycoprotein to the Cell Surface, Indicating Enhanced Cholesterol Trafficking in MDR Cells, Which Makes Them Resistant to Cholesterol Modifications
- PMID: 37569709
- PMCID: PMC10419235
- DOI: 10.3390/ijms241512335
Cholesterol-Depletion-Induced Membrane Repair Carries a Raft Conformer of P-Glycoprotein to the Cell Surface, Indicating Enhanced Cholesterol Trafficking in MDR Cells, Which Makes Them Resistant to Cholesterol Modifications
Abstract
The human P-glycoprotein (P-gp), a transporter responsible for multidrug resistance, is present in the plasma membrane's raft and non-raft domains. One specific conformation of P-gp that binds to the monoclonal antibody UIC2 is primarily associated with raft domains and displays heightened internalization in cells overexpressing P-gp, such as in NIH-3T3 MDR1 cells. Our primary objective was to investigate whether the trafficking of this particular P-gp conformer is dependent on cholesterol levels. Surprisingly, depleting cholesterol using cyclodextrin resulted in an unexpected increase in the proportion of raft-associated P-gp within the cell membrane, as determined by UIC2-reactive P-gp. This increase appears to be a compensatory response to cholesterol loss from the plasma membrane, whereby cholesterol-rich raft micro-domains are delivered to the cell surface through an augmented exocytosis process. Furthermore, this exocytotic event is found to be part of a complex trafficking mechanism involving lysosomal exocytosis, which contributes to membrane repair after cholesterol reduction induced by cyclodextrin treatment. Notably, cells overexpressing P-gp demonstrated higher total cellular cholesterol levels, an increased abundance of stable lysosomes, and more effective membrane repair following cholesterol modifications. These modifications encompassed exocytotic events that involved the transport of P-gp-carrying rafts. Importantly, the enhanced membrane repair capability resulted in a durable phenotype for MDR1 expressing cells, as evidenced by significantly improved viabilities of multidrug-resistant Pgp-overexpressing immortal NIH-3T3 MDR1 and MDCK-MDR1 cells compared to their parents when subjected to cholesterol alterations.
Keywords: ABCB1 transporter; UIC2; cyclodextrin; membrane repair; raft; trafficking.
Conflict of interest statement
The authors declare no conflict of interest.
Figures
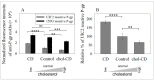
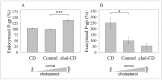
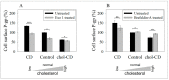
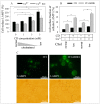
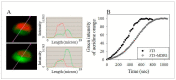
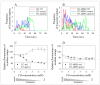
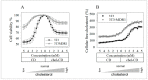
Similar articles
-
Raft and cytoskeleton associations of an ABC transporter: P-glycoprotein.Cytometry A. 2004 Oct;61(2):105-16. doi: 10.1002/cyto.a.20081. Cytometry A. 2004. PMID: 15382145
-
An anti-CD19 antibody inhibits the interaction between P-glycoprotein (P-gp) and CD19, causes P-gp to translocate out of lipid rafts, and chemosensitizes a multidrug-resistant (MDR) lymphoma cell line.Blood. 2004 Jul 1;104(1):178-83. doi: 10.1182/blood-2003-12-4255. Epub 2004 Mar 4. Blood. 2004. PMID: 15001473
-
Raltegravir does not revert efflux activity of MDR1-P-glycoprotein in human MDR cells.BMC Pharmacol Toxicol. 2013 Sep 20;14:47. doi: 10.1186/2050-6511-14-47. BMC Pharmacol Toxicol. 2013. PMID: 24053678 Free PMC article.
-
P-glycoprotein and 'lipid rafts': some ambiguous mutual relationships (floating on them, building them or meeting them by chance?).Cell Mol Life Sci. 2006 May;63(9):1038-59. doi: 10.1007/s00018-005-5554-9. Cell Mol Life Sci. 2006. PMID: 16721513 Free PMC article. Review.
-
Structure, function and regulation of P-glycoprotein and its clinical relevance in drug disposition.Xenobiotica. 2008 Jul;38(7-8):802-32. doi: 10.1080/00498250701867889. Xenobiotica. 2008. PMID: 18668431 Review.
Cited by
-
Quercetin represses cholesterol metabolism and mitigates resistance to cisplatin in oral squamous cell carcinoma by regulating AGR2/AKT/SREBP2 axis.Heliyon. 2024 Sep 11;10(18):e37518. doi: 10.1016/j.heliyon.2024.e37518. eCollection 2024 Sep 30. Heliyon. 2024. PMID: 39323844 Free PMC article.
References
-
- Cho W., Ralko A., Sharma A. An In Situ Fluorescence Assay for Cholesterol Transporter Activity of the Patched. Methods Mol. Biol. 2022;2374:37–47. - PubMed
MeSH terms
Substances
Grants and funding
LinkOut - more resources
Full Text Sources
Miscellaneous