Gating of β-Barrel Protein Pores, Porins, and Channels: An Old Problem with New Facets
- PMID: 37569469
- PMCID: PMC10418385
- DOI: 10.3390/ijms241512095
Gating of β-Barrel Protein Pores, Porins, and Channels: An Old Problem with New Facets
Abstract
β barrels are ubiquitous proteins in the outer membranes of mitochondria, chloroplasts, and Gram-negative bacteria. These transmembrane proteins (TMPs) execute a wide variety of tasks. For example, they can serve as transporters, receptors, membrane-bound enzymes, as well as adhesion, structural, and signaling elements. In addition, multimeric β barrels are common structural scaffolds among many pore-forming toxins. Significant progress has been made in understanding the functional, structural, biochemical, and biophysical features of these robust and versatile proteins. One frequently encountered fundamental trait of all β barrels is their voltage-dependent gating. This process consists of reversible or permanent conformational transitions between a large-conductance, highly permeable open state and a low-conductance, solute-restrictive closed state. Several intrinsic molecular mechanisms and environmental factors modulate this universal property of β barrels. This review article outlines the typical signatures of voltage-dependent gating. Moreover, we discuss recent developments leading to a better qualitative understanding of the closure dynamics of these TMPs.
Keywords: conformational transitions; electrophysiology; membrane proteins; protein folding; single-molecule dynamics.
Conflict of interest statement
The authors declare no competing interest.
Figures
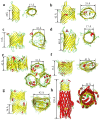
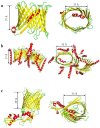
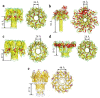
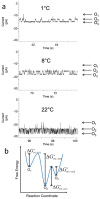
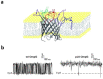
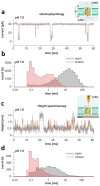
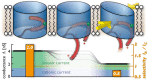
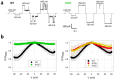
Similar articles
-
Emerging issues of connexin channels: biophysics fills the gap.Q Rev Biophys. 2001 Aug;34(3):325-472. doi: 10.1017/s0033583501003705. Q Rev Biophys. 2001. PMID: 11838236 Review.
-
Voltage gating is a fundamental feature of porin and toxin beta-barrel membrane channels.FEBS Lett. 1998 Jul 24;431(3):305-8. doi: 10.1016/s0014-5793(98)00761-3. FEBS Lett. 1998. PMID: 9714531 Review.
-
Identification and Characterization of the Major Porin of Desulfovibrio vulgaris Hildenborough.J Bacteriol. 2017 Oct 31;199(23):e00286-17. doi: 10.1128/JB.00286-17. Print 2017 Dec 1. J Bacteriol. 2017. PMID: 28874410 Free PMC article.
-
The structural biology of β-barrel membrane proteins: a summary of recent reports.Curr Opin Struct Biol. 2011 Aug;21(4):523-31. doi: 10.1016/j.sbi.2011.05.005. Epub 2011 Jun 28. Curr Opin Struct Biol. 2011. PMID: 21719274 Free PMC article. Review.
-
Mechanisms of solute transport through outer membrane porins: burning down the house.Curr Opin Microbiol. 1998 Apr;1(2):238-47. doi: 10.1016/s1369-5274(98)80017-9. Curr Opin Microbiol. 1998. PMID: 10066479 Review.
Cited by
-
Beta-Barrel Channel Response to High Electric Fields: Functional Gating or Reversible Denaturation?Int J Mol Sci. 2023 Nov 23;24(23):16655. doi: 10.3390/ijms242316655. Int J Mol Sci. 2023. PMID: 38068977 Free PMC article.
-
Beta-Barrel Nanopores as Diagnostic Sensors: An Engineering Perspective.Biosensors (Basel). 2024 Jul 16;14(7):345. doi: 10.3390/bios14070345. Biosensors (Basel). 2024. PMID: 39056622 Free PMC article. Review.
References
Publication types
MeSH terms
Substances
Grants and funding
LinkOut - more resources
Full Text Sources