Cardiomyocyte external mechanical unloading activates modifications of α-actinin differently from sarcomere-originated unloading
- PMID: 37551968
- PMCID: PMC11285078
- DOI: 10.1111/febs.16925
Cardiomyocyte external mechanical unloading activates modifications of α-actinin differently from sarcomere-originated unloading
Abstract
Loss of myocardial mass in a neonatal rat cardiomyocyte culture is studied to determine whether there is a distinguishable cellular response based on the origin of mechano-signals. The approach herein compares the sarcomeric assembly and disassembly processes in heart cells by imposing mechano-signals at the interface with the extracellular matrix (extrinsic) and at the level of the myofilaments (intrinsic). Experiments compared the effects of imposed internal (inside/out) and external (outside/in) loading and unloading on modifications in neonatal rat cardiomyocytes. Unloading of the cellular substrate by myosin inhibition (1 μm mavacamten), or cessation of cyclic strain (1 Hz, 10% strain) after preconditioning, led to significant disassembly of sarcomeric α-actinin by 6 h. In myosin inhibition, this was accompanied by redistribution of intracellular poly-ubiquitin K48 to the cellular periphery relative to the poly-ubiquitin K48 reservoir at the I-band. Moreover, loading and unloading of the cellular substrate led to a three-fold increase in post-translational modifications (PTMs) when compared to the myosin-specific activation or inhibition. Specifically, phosphorylation increased with loading while ubiquitination increased with unloading, which may involve extracellular signal-regulated kinase 1/2 and focal adhesion kinase activation. The identified PTMs, including ubiquitination, acetylation, and phosphorylation, are proposed to modify internal domains in α-actinin to increase its propensity to bind F-actin. These results demonstrate a link between mechanical feedback and sarcomere protein homeostasis via PTMs of α-actinin that exemplify how cardiomyocytes exhibit differential responses to the origin of force. The implications of sarcomere regulation governed by PTMs of α-actinin are discussed with respect to cardiac atrophy and heart failure.
Keywords: HCM; HFrEF; calponin homology domain; myotrope; sarcopenia.
© 2023 The Authors. The FEBS Journal published by John Wiley & Sons Ltd on behalf of Federation of European Biochemical Societies.
Conflict of interest statement
Conflict of interest
RJS is a member of the scientific advisory board of Cytokinetics and a consultant to Pfizer and Edgewise Therapeutics. The remaining authors declare no competing financial interests.
Figures
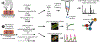
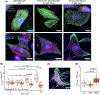
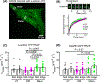
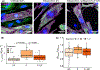
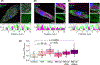
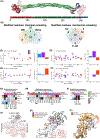
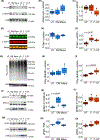
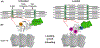
Similar articles
-
Dynamic Alterations to α-Actinin Accompanying Sarcomere Disassembly and Reassembly during Cardiomyocyte Mitosis.PLoS One. 2015 Jun 15;10(6):e0129176. doi: 10.1371/journal.pone.0129176. eCollection 2015. PLoS One. 2015. PMID: 26076379 Free PMC article.
-
Force Generation via β-Cardiac Myosin, Titin, and α-Actinin Drives Cardiac Sarcomere Assembly from Cell-Matrix Adhesions.Dev Cell. 2018 Jan 8;44(1):87-96.e5. doi: 10.1016/j.devcel.2017.12.012. Epub 2018 Jan 8. Dev Cell. 2018. PMID: 29316444 Free PMC article.
-
Cyclic mechanical strain of myocytes modifies CapZβ1 post translationally via PKCε.J Muscle Res Cell Motil. 2015 Oct;36(4-5):329-37. doi: 10.1007/s10974-015-9420-6. Epub 2015 Oct 1. J Muscle Res Cell Motil. 2015. PMID: 26429793 Free PMC article.
-
SUMO system - a key regulator in sarcomere organization.FEBS J. 2020 Jun;287(11):2176-2190. doi: 10.1111/febs.15263. Epub 2020 Mar 13. FEBS J. 2020. PMID: 32096922 Review.
-
Role of c-Src in cellular events associated with colony-stimulating factor-1-induced spreading in osteoclasts.Mol Reprod Dev. 1997 Jan;46(1):104-8. doi: 10.1002/(SICI)1098-2795(199701)46:1<104::AID-MRD16>3.0.CO;2-2. Mol Reprod Dev. 1997. PMID: 8981371 Review.
Cited by
-
Modulating cardiac physiology in engineered heart tissue with the bidirectional optogenetic tool BiPOLES.Pflugers Arch. 2023 Dec;475(12):1463-1477. doi: 10.1007/s00424-023-02869-x. Epub 2023 Oct 21. Pflugers Arch. 2023. PMID: 37863976 Free PMC article.
-
Emerging Concepts of Mechanisms Controlling Cardiac Tension: Focus on Familial Dilated Cardiomyopathy (DCM) and Sarcomere-Directed Therapies.Biomedicines. 2024 May 2;12(5):999. doi: 10.3390/biomedicines12050999. Biomedicines. 2024. PMID: 38790961 Free PMC article. Review.
References
-
- Kim GH, Uriel N & Burkhoff D (2018) Reverse remodelling and myocardial recovery in heart failure. Nat Rev Cardiol 15, 83–96. - PubMed
Publication types
MeSH terms
Substances
Associated data
- Actions
Grants and funding
LinkOut - more resources
Full Text Sources
Miscellaneous