Assessing the Impact of Polyethylene Nano/Microplastic Exposure on Human Vaginal Keratinocytes
- PMID: 37511139
- PMCID: PMC10380279
- DOI: 10.3390/ijms241411379
Assessing the Impact of Polyethylene Nano/Microplastic Exposure on Human Vaginal Keratinocytes
Abstract
The global rise of single-use throw-away plastic products has elicited a massive increase in the nano/microplastics (N/MPLs) exposure burden in humans. Recently, it has been demonstrated that disposable period products may release N/MPLs with usage, which represents a potential threat to women's health which has not been scientifically addressed yet. By using polyethyl ene (PE) particles (200 nm to 9 μm), we showed that acute exposure to a high concentration of N/MPLs induced cell toxicity in vaginal keratinocytes after effective cellular uptake, as viability and apoptosis data suggest, along with transmission electron microscopy (TEM) observations. The internalised N/MPLs altered the expression of junctional and adherence proteins and the organisation of the actin cortex, influencing the level of genes involved in oxidative stress signalling pathways and that of miRNAs related to epithelial barrier function. When the exposure to PE N/MPLs was discontinued or became chronic, cells were able to recover from the negative effects on viability and differentiation/proliferation gene expression in a few days. However, in all cases, PE N/MPL exposure prompted a sustained alteration of DNA methyltransferase and DNA demethylase expression, which might impact epigenetic regulation processes, leading to accelerated cell ageing and inflammation, or the occurrence of malignant transformation.
Keywords: microplastics; nano/microparticles uptake; nanoplastics; period products; polyethylene; vaginal keratinocytes.
Conflict of interest statement
The authors declare no conflict of interest.
Figures
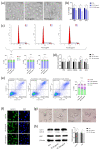
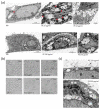
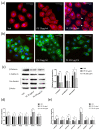
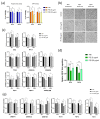
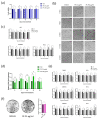
Similar articles
-
Combined exposure of the bivalve Mytilus galloprovincialis to polyethylene microplastics and two pharmaceuticals (citalopram and bezafibrate): Bioaccumulation and metabolomic studies.J Hazard Mater. 2023 Sep 15;458:131904. doi: 10.1016/j.jhazmat.2023.131904. Epub 2023 Jun 21. J Hazard Mater. 2023. PMID: 37356174
-
Hematological consequences of polyethylene microplastics toxicity in male rats: Oxidative stress, genetic, and epigenetic links.Toxicology. 2023 Jun 15;492:153545. doi: 10.1016/j.tox.2023.153545. Epub 2023 May 9. Toxicology. 2023. PMID: 37169321
-
Micro-sized polyethylene particles affect cell viability and oxidative stress responses in human colorectal adenocarcinoma Caco-2 and HT-29 cells.Sci Total Environ. 2023 Apr 1;867:161512. doi: 10.1016/j.scitotenv.2023.161512. Epub 2023 Jan 7. Sci Total Environ. 2023. PMID: 36626990
-
Potential lifetime effects caused by cellular uptake of nanoplastics: A review.Environ Pollut. 2023 Jul 15;329:121668. doi: 10.1016/j.envpol.2023.121668. Epub 2023 Apr 20. Environ Pollut. 2023. PMID: 37087090 Review.
-
Atmospheric microplastic and nanoplastic: The toxicological paradigm on the cellular system.Ecotoxicol Environ Saf. 2023 Jul 1;259:115018. doi: 10.1016/j.ecoenv.2023.115018. Epub 2023 May 20. Ecotoxicol Environ Saf. 2023. PMID: 37216859 Review.
Cited by
-
Microplastics in the Human Body: Exposure, Detection, and Risk of Carcinogenesis: A State-of-the-Art Review.Cancers (Basel). 2024 Nov 1;16(21):3703. doi: 10.3390/cancers16213703. Cancers (Basel). 2024. PMID: 39518141 Free PMC article. Review.
-
Antitumour effects of SFX-01 molecule in combination with ionizing radiation in preclinical and in vivo models of rhabdomyosarcoma.BMC Cancer. 2024 Jul 8;24(1):814. doi: 10.1186/s12885-024-12536-8. BMC Cancer. 2024. PMID: 38977944 Free PMC article.
-
Health Implications of Widespread Micro- and Nanoplastic Exposure: Environmental Prevalence, Mechanisms, and Biological Impact on Humans.Toxics. 2024 Oct 10;12(10):730. doi: 10.3390/toxics12100730. Toxics. 2024. PMID: 39453150 Free PMC article. Review.
-
Micro(nano)plastics: an Emerging Burden for Human Health.Int J Biol Sci. 2024 Oct 21;20(14):5779-5792. doi: 10.7150/ijbs.99556. eCollection 2024. Int J Biol Sci. 2024. PMID: 39494332 Free PMC article. Review.
-
Adverse Effects of Micro- and Nanoplastics on Humans and the Environment.Int J Mol Sci. 2023 Oct 31;24(21):15822. doi: 10.3390/ijms242115822. Int J Mol Sci. 2023. PMID: 37958802 Free PMC article.
References
-
- Hartmann N.B., Hüffer T., Thompson R.C., Hassellöv M., Verschoor A., Daugaard A.E., Rist S., Karlsson T., Brennholt N., Cole M., et al. Are We Speaking the Same Language? Recommendations for a Definition and Categorization Framework for Plastic Debris. Environ. Sci. Technol. 2019;53:1039–1047. doi: 10.1021/acs.est.8b05297. - DOI - PubMed
-
- Park H., Park B. Review of Microplastic Distribution, Toxicity, Analysis Methods, and Removal Technologies. Water. 2021;13:2736. doi: 10.3390/w13192736. - DOI
MeSH terms
Substances
Grants and funding
LinkOut - more resources
Full Text Sources