Voltage-Gated Proton Channels in the Tree of Life
- PMID: 37509071
- PMCID: PMC10377628
- DOI: 10.3390/biom13071035
Voltage-Gated Proton Channels in the Tree of Life
Abstract
With a single gene encoding HV1 channel, proton channel diversity is particularly low in mammals compared to other members of the superfamily of voltage-gated ion channels. Nonetheless, mammalian HV1 channels are expressed in many different tissues and cell types where they exert various functions. In the first part of this review, we regard novel aspects of the functional expression of HV1 channels in mammals by differentially comparing their involvement in (1) close conjunction with the NADPH oxidase complex responsible for the respiratory burst of phagocytes, and (2) in respiratory burst independent functions such as pH homeostasis or acid extrusion. In the second part, we dissect expression of HV channels within the eukaryotic tree of life, revealing the immense diversity of the channel in other phylae, such as mollusks or dinoflagellates, where several genes encoding HV channels can be found within a single species. In the last part, a comprehensive overview of the biophysical properties of a set of twenty different HV channels characterized electrophysiologically, from Mammalia to unicellular protists, is given.
Keywords: Aplysia; Ecdysozoa; Lophotrochozoa; NADPH oxidase; coccolithophores; insect; mollusks; pH-dependent gating; voltage-gated proton channel; voltage-sensing.
Conflict of interest statement
The authors declare no conflict of interest.
Figures

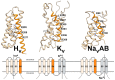
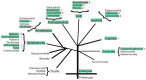
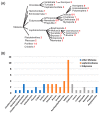
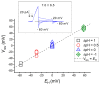
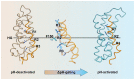
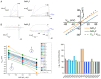
Similar articles
-
Voltage-gated proton channels.Compr Physiol. 2012 Apr;2(2):1355-85. doi: 10.1002/cphy.c100071. Compr Physiol. 2012. PMID: 23798303 Free PMC article. Review.
-
Identification of an HV 1 voltage-gated proton channel in insects.FEBS J. 2016 Apr;283(8):1453-64. doi: 10.1111/febs.13680. Epub 2016 Mar 6. FEBS J. 2016. PMID: 26866814
-
Unexpected expansion of the voltage-gated proton channel family.FEBS J. 2023 Feb;290(4):1008-1026. doi: 10.1111/febs.16617. Epub 2022 Sep 20. FEBS J. 2023. PMID: 36062330 Free PMC article.
-
Voltage-gated proton channels find their dream job managing the respiratory burst in phagocytes.Physiology (Bethesda). 2010 Feb;25(1):27-40. doi: 10.1152/physiol.00039.2009. Physiology (Bethesda). 2010. PMID: 20134026 Free PMC article. Review.
-
Role of the Voltage-Gated Proton Channel Hv1 in Nervous Systems.Neurosci Bull. 2023 Jul;39(7):1157-1172. doi: 10.1007/s12264-023-01053-6. Epub 2023 Apr 8. Neurosci Bull. 2023. PMID: 37029856 Free PMC article. Review.
Cited by
-
Identification of a Novel Structural Class of HV1 Inhibitors by Structure-Based Virtual Screening.J Chem Inf Model. 2024 Jun 24;64(12):4850-4862. doi: 10.1021/acs.jcim.4c00240. Epub 2024 Jun 8. J Chem Inf Model. 2024. PMID: 38850237 Free PMC article.
-
The Emerging Role of Microglial Hv1 as a Target for Immunomodulation in Myelin Repair.Aging Dis. 2024 May 7;15(3):1176-1203. doi: 10.14336/AD.2023.1107. Aging Dis. 2024. PMID: 38029392 Free PMC article. Review.
References
-
- Baldridge C.W., Gerard R.W. The extra respiration of phagocytosis. Am. J. Physiol. Content. 1932;103:235–236. doi: 10.1152/ajplegacy.1932.103.1.235. - DOI
Publication types
MeSH terms
Substances
Grants and funding
LinkOut - more resources
Full Text Sources