Characterising the RNA-binding protein atlas of the mammalian brain uncovers RBM5 misregulation in mouse models of Huntington's disease
- PMID: 37468457
- PMCID: PMC10356804
- DOI: 10.1038/s41467-023-39936-x
Characterising the RNA-binding protein atlas of the mammalian brain uncovers RBM5 misregulation in mouse models of Huntington's disease
Abstract
RNA-binding proteins (RBPs) are key players regulating RNA processing and are associated with disorders ranging from cancer to neurodegeneration. Here, we present a proteomics workflow for large-scale identification of RBPs and their RNA-binding regions in the mammalian brain identifying 526 RBPs. Analysing brain tissue from males of the Huntington's disease (HD) R6/2 mouse model uncovered differential RNA-binding of the alternative splicing regulator RBM5. Combining several omics workflows, we show that RBM5 binds differentially to transcripts enriched in pathways of neurodegeneration in R6/2 brain tissue. We further find these transcripts to undergo changes in splicing and demonstrate that RBM5 directly regulates these changes in human neurons derived from embryonic stem cells. Finally, we reveal that RBM5 interacts differently with several known huntingtin interactors and components of huntingtin aggregates. Collectively, we demonstrate the applicability of our method for capturing RNA interactor dynamics in the contexts of tissue and disease.
© 2023. The Author(s).
Conflict of interest statement
The authors declare no competing interests.
Figures
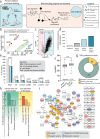
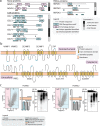
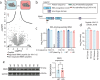
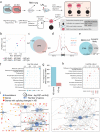
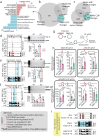
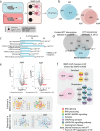
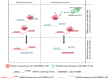
Similar articles
-
Downregulation of glial genes involved in synaptic function mitigates Huntington's disease pathogenesis.Elife. 2021 Apr 19;10:e64564. doi: 10.7554/eLife.64564. Elife. 2021. PMID: 33871358 Free PMC article.
-
The nuclear splicing factor RNA binding motif 5 promotes caspase activation in human neuronal cells, and increases after traumatic brain injury in mice.J Cereb Blood Flow Metab. 2015 Mar 31;35(4):655-66. doi: 10.1038/jcbfm.2014.242. J Cereb Blood Flow Metab. 2015. PMID: 25586139 Free PMC article.
-
RBM5 is a male germ cell splicing factor and is required for spermatid differentiation and male fertility.PLoS Genet. 2013;9(7):e1003628. doi: 10.1371/journal.pgen.1003628. Epub 2013 Jul 25. PLoS Genet. 2013. PMID: 23935508 Free PMC article.
-
Implications of Tau Dysregulation in Huntington's Disease and Potential for New Therapeutics.J Huntingtons Dis. 2023;12(1):1-13. doi: 10.3233/JHD-230569. J Huntingtons Dis. 2023. PMID: 37092231 Free PMC article. Review.
-
Mouse Models of Huntington's Disease.Methods Mol Biol. 2018;1780:97-120. doi: 10.1007/978-1-4939-7825-0_6. Methods Mol Biol. 2018. PMID: 29856016 Review.
Cited by
-
RNA-binding protein RBM5 plays an essential role in acute myeloid leukemia by activating the oncogenic protein HOXA9.Genome Biol. 2024 Jan 12;25(1):16. doi: 10.1186/s13059-023-03149-8. Genome Biol. 2024. PMID: 38216972 Free PMC article.
-
Gene knockout of RNA binding motif 5 in the brain alters RIMS2 protein homeostasis in the cerebellum and Hippocampus and exacerbates behavioral deficits after a TBI in mice.Exp Neurol. 2024 Apr;374:114690. doi: 10.1016/j.expneurol.2024.114690. Epub 2024 Jan 12. Exp Neurol. 2024. PMID: 38218585
-
High-sensitivity in situ capture of endogenous RNA-protein interactions in fixed cells and primary tissues.Nat Commun. 2024 Aug 16;15(1):7067. doi: 10.1038/s41467-024-50363-4. Nat Commun. 2024. PMID: 39152130 Free PMC article.
-
Alternative splicing and related RNA binding proteins in human health and disease.Signal Transduct Target Ther. 2024 Feb 2;9(1):26. doi: 10.1038/s41392-024-01734-2. Signal Transduct Target Ther. 2024. PMID: 38302461 Free PMC article. Review.
References
Publication types
MeSH terms
Substances
LinkOut - more resources
Full Text Sources
Medical
Molecular Biology Databases