Formation of nuclear CPSF6/CPSF5 biomolecular condensates upon HIV-1 entry into the nucleus is important for productive infection
- PMID: 37414787
- PMCID: PMC10325960
- DOI: 10.1038/s41598-023-37364-x
Formation of nuclear CPSF6/CPSF5 biomolecular condensates upon HIV-1 entry into the nucleus is important for productive infection
Abstract
The early events of HIV-1 infection involve the transport of the viral core into the nucleus. This event triggers the translocation of CPSF6 from paraspeckles into nuclear speckles forming puncta-like structures. Our investigations revealed that neither HIV-1 integration nor reverse transcription is required for the formation of puncta-like structures. Moreover, HIV-1 viruses without viral genome are competent for the induction of CPSF6 puncta-like structures. In agreement with the notion that HIV-1 induced CPSF6 puncta-like structures are biomolecular condensates, we showed that osmotic stress and 1,6-hexanediol induced the disassembly of CPSF6 condensates. Interestingly, replacing the osmotic stress by isotonic media re-assemble CPSF6 condensates in the cytoplasm of the cell. To test whether CPSF6 condensates were important for infection we utilized hypertonic stress, which prevents formation of CPSF6 condensates, during infection. Remarkably, preventing the formation of CPSF6 condensates inhibits the infection of wild type HIV-1 but not of HIV-1 viruses bearing the capsid changes N74D and A77V, which do not form CPSF6 condensates during infection1,2. We also investigated whether the functional partners of CPSF6 are recruited to the condensates upon infection. Our experiments revealed that CPSF5, but not CPSF7, co-localized with CPSF6 upon HIV-1 infection. We found condensates containing CPSF6/CPSF5 in human T cells and human primary macrophages upon HIV-1 infection. Additionally, we observed that the integration cofactor LEDGF/p75 changes distribution upon HIV-1 infection and surrounds the CPSF6/CPSF5 condensates. Overall, our work demonstrated that CPSF6 and CPSF5 are forming biomolecular condensates that are important for infection of wild type HIV-1 viruses.
© 2023. The Author(s).
Conflict of interest statement
The authors declare no competing interests.
Figures
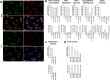
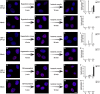
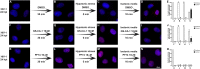
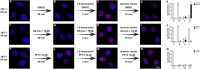
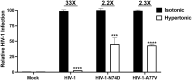
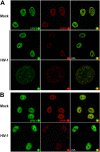
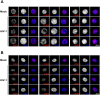
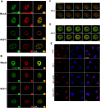
Similar articles
-
Detection of CPSF6 in Biomolecular Condensates as a Reporter of HIV-1 Nuclear Import.Methods Mol Biol. 2024;2807:127-138. doi: 10.1007/978-1-0716-3862-0_9. Methods Mol Biol. 2024. PMID: 38743225
-
HIV-1 Capsid Rapidly Induces Long-Lived CPSF6 Puncta in Non-Dividing Cells, but Similar Puncta Already Exist in Uninfected T-Cells.Viruses. 2024 Apr 25;16(5):670. doi: 10.3390/v16050670. Viruses. 2024. PMID: 38793552 Free PMC article.
-
HIV-1-induced translocation of CPSF6 to biomolecular condensates.Trends Microbiol. 2024 Aug;32(8):781-790. doi: 10.1016/j.tim.2024.01.001. Epub 2024 Jan 23. Trends Microbiol. 2024. PMID: 38267295 Review.
-
The Cleavage and Polyadenylation Specificity Factor 6 (CPSF6) Subunit of the Capsid-recruited Pre-messenger RNA Cleavage Factor I (CFIm) Complex Mediates HIV-1 Integration into Genes.J Biol Chem. 2016 May 27;291(22):11809-19. doi: 10.1074/jbc.M116.721647. Epub 2016 Mar 18. J Biol Chem. 2016. PMID: 26994143 Free PMC article.
-
HIV-Induced CPSF6 Condensates.J Mol Biol. 2023 Aug 15;435(16):168094. doi: 10.1016/j.jmb.2023.168094. Epub 2023 Apr 14. J Mol Biol. 2023. PMID: 37061085 Review.
Cited by
-
Detection of CPSF6 in Biomolecular Condensates as a Reporter of HIV-1 Nuclear Import.Methods Mol Biol. 2024;2807:127-138. doi: 10.1007/978-1-0716-3862-0_9. Methods Mol Biol. 2024. PMID: 38743225
-
HIV-1 Capsid Rapidly Induces Long-Lived CPSF6 Puncta in Non-Dividing Cells, but Similar Puncta Already Exist in Uninfected T-Cells.Viruses. 2024 Apr 25;16(5):670. doi: 10.3390/v16050670. Viruses. 2024. PMID: 38793552 Free PMC article.
-
The nuclear localization signal of CPSF6 governs post-nuclear import steps of HIV-1 infection.bioRxiv [Preprint]. 2024 Jun 24:2024.06.20.599834. doi: 10.1101/2024.06.20.599834. bioRxiv. 2024. PMID: 38979149 Free PMC article. Preprint.
-
HIV-1 usurps mixed-charge domain-dependent CPSF6 phase separation for higher-order capsid binding, nuclear entry and viral DNA integration.Nucleic Acids Res. 2024 Oct 14;52(18):11060-11082. doi: 10.1093/nar/gkae769. Nucleic Acids Res. 2024. PMID: 39258548 Free PMC article.
-
HIV-1-induced translocation of CPSF6 to biomolecular condensates.Trends Microbiol. 2024 Aug;32(8):781-790. doi: 10.1016/j.tim.2024.01.001. Epub 2024 Jan 23. Trends Microbiol. 2024. PMID: 38267295 Review.
References
Publication types
MeSH terms
Substances
Grants and funding
LinkOut - more resources
Full Text Sources
Medical
Research Materials