Alteration of replication protein A binding mode on single-stranded DNA by NSMF potentiates RPA phosphorylation by ATR kinase
- PMID: 37378431
- PMCID: PMC10450186
- DOI: 10.1093/nar/gkad543
Alteration of replication protein A binding mode on single-stranded DNA by NSMF potentiates RPA phosphorylation by ATR kinase
Abstract
Replication protein A (RPA), a eukaryotic single-stranded DNA (ssDNA) binding protein, dynamically interacts with ssDNA in different binding modes and plays essential roles in DNA metabolism such as replication, repair, and recombination. RPA accumulation on ssDNA due to replication stress triggers the DNA damage response (DDR) by activating the ataxia telangiectasia and RAD3-related (ATR) kinase, which phosphorylates itself and downstream DDR factors, including RPA. We recently reported that the N-methyl-D-aspartate receptor synaptonuclear signaling and neuronal migration factor (NSMF), a neuronal protein associated with Kallmann syndrome, promotes RPA32 phosphorylation via ATR upon replication stress. However, how NSMF enhances ATR-mediated RPA32 phosphorylation remains elusive. Here, we demonstrate that NSMF colocalizes and physically interacts with RPA at DNA damage sites in vivo and in vitro. Using purified RPA and NSMF in biochemical and single-molecule assays, we find that NSMF selectively displaces RPA in the more weakly bound 8- and 20-nucleotide binding modes from ssDNA, allowing the retention of more stable RPA molecules in the 30-nt binding mode. The 30-nt binding mode of RPA enhances RPA32 phosphorylation by ATR, and phosphorylated RPA becomes stabilized on ssDNA. Our findings provide new mechanistic insight into how NSMF facilitates the role of RPA in the ATR pathway.
© The Author(s) 2023. Published by Oxford University Press on behalf of Nucleic Acids Research.
Figures
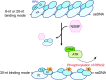
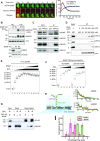
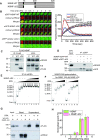
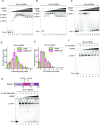
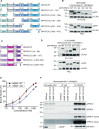
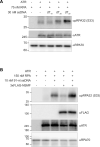
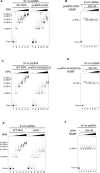
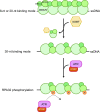
Similar articles
-
Phosphorylation of replication protein A by S-phase checkpoint kinases.DNA Repair (Amst). 2006 Mar 7;5(3):369-80. doi: 10.1016/j.dnarep.2005.11.007. Epub 2006 Jan 18. DNA Repair (Amst). 2006. PMID: 16412704
-
Distinct roles for DNA-PK, ATM and ATR in RPA phosphorylation and checkpoint activation in response to replication stress.Nucleic Acids Res. 2012 Nov;40(21):10780-94. doi: 10.1093/nar/gks849. Epub 2012 Sep 12. Nucleic Acids Res. 2012. PMID: 22977173 Free PMC article.
-
Sensing DNA damage through ATRIP recognition of RPA-ssDNA complexes.Science. 2003 Jun 6;300(5625):1542-8. doi: 10.1126/science.1083430. Science. 2003. PMID: 12791985
-
Activation of ATR-related protein kinase upon DNA damage recognition.Curr Genet. 2020 Apr;66(2):327-333. doi: 10.1007/s00294-019-01039-w. Epub 2019 Oct 17. Curr Genet. 2020. PMID: 31624858 Free PMC article. Review.
-
Functions of human replication protein A (RPA): from DNA replication to DNA damage and stress responses.J Cell Physiol. 2006 Aug;208(2):267-73. doi: 10.1002/jcp.20622. J Cell Physiol. 2006. PMID: 16523492 Free PMC article. Review.
Cited by
-
The Intriguing Mystery of RPA Phosphorylation in DNA Double-Strand Break Repair.Genes (Basel). 2024 Jan 27;15(2):167. doi: 10.3390/genes15020167. Genes (Basel). 2024. PMID: 38397158 Free PMC article. Review.
-
Direct visualization of replication and R-loop collision using single-molecule imaging.Nucleic Acids Res. 2024 Jan 11;52(1):259-273. doi: 10.1093/nar/gkad1101. Nucleic Acids Res. 2024. PMID: 37994723 Free PMC article.
References
-
- Wold M.S. Replication protein A: a heterotrimeric, single-stranded DNA-binding protein required for eukaryotic DNA metabolism. Annu. Rev. Biochem. 1997; 66:61–92. - PubMed
-
- Iftode C., Daniely Y., Borowiec J.A.. Replication protein A (RPA): the eukaryotic SSB. Crit. Rev. Biochem. Mol. 1999; 34:141–180. - PubMed
Publication types
MeSH terms
Substances
LinkOut - more resources
Full Text Sources
Research Materials
Miscellaneous