Membrane-destabilizing ionizable lipid empowered imaging-guided siRNA delivery and cancer treatment
- PMID: 37366466
- PMCID: PMC10291568
- DOI: 10.1002/EXP.20210008
Membrane-destabilizing ionizable lipid empowered imaging-guided siRNA delivery and cancer treatment
Abstract
One of the imperative medical requirements for cancer treatment is how to establish an imaging-guided nanocarrier that combines therapeutic and imaging agents into one system. siRNA therapeutics have shown promising prospects in controlling life-threatening diseases. However, it is still challenging to develop siRNA formulations with excellent cellular entry capability, efficient endosomal escape, and simultaneous visualization. Herein, we fabricated multifunctional ionizable lipid nanoparticles (iLNPs) for targeted delivery of siRNA and MRI contrast agent. The iLNPs comprises DSPC, cholesterol, PEGylated lipid, contrast agent DTPA-BSA (Gd), and ionizable lipid termed iBL0104. siRNA-loaded iLNPs (iLNPs/siRNA) could be decorated with a tumor targeting cyclic peptide (c(GRGDSPKC)) (termed GARP), or without targeting modification (termed GAP). Data revealed that GARP/siRNA iLNPs exhibited significantly higher cellular entry efficiency than GAP/siRNA iLNPs. GARP/siRNA iLNPs rapidly and effectively escaped from endosome and lysosome after internalization. Compared with GAP/siPLK1, GARP/siPLK1 exhibited better tumor inhibition efficacy in both cell-line derived xenograft and liver cancer patient derived xenograft murine models. In addition, GARP formulation displayed ideal MRI effect in tumor-bearing mice, and was well tolerated by testing animals. Therefore, this study provides an excellent example for achieving imaging-guided and tumor-targeted siRNA delivery and cancer treatment, highlighting its promising potential for translational medicine application.
Keywords: MRI; cancer treatment; hepatocellular carcinoma; lipid nanoparticle; lipid‐like material; siRNA.
© 2021 The Authors. Exploration published by Henan University and John Wiley & Sons Australia, Ltd.
Conflict of interest statement
S.G. and Y.H. declare that a patent relative the study has been filed. The remaining authors declare no competing interests.
Figures
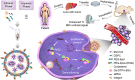
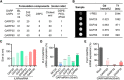
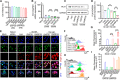
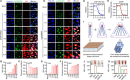
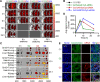
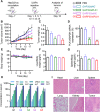
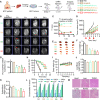
Similar articles
-
Polysorbate 80-containing ionizable lipid nanoparticles for mRNA delivery.Biomater Sci. 2024 Oct 22;12(21):5573-5581. doi: 10.1039/d4bm00523f. Biomater Sci. 2024. PMID: 39297400
-
mRNA Synthesis and Encapsulation in Ionizable Lipid Nanoparticles.Curr Protoc. 2023 Sep;3(9):e898. doi: 10.1002/cpz1.898. Curr Protoc. 2023. PMID: 37747354
-
Engineered ionizable lipid siRNA conjugates enhance endosomal escape but induce toxicity in vivo.J Control Release. 2022 Sep;349:831-843. doi: 10.1016/j.jconrel.2022.07.041. Epub 2022 Aug 3. J Control Release. 2022. PMID: 35917865 Free PMC article.
-
The development of highly dense highly protected surfactant ionizable lipid RNA loaded nanoparticles.Front Immunol. 2023 Feb 27;14:1129296. doi: 10.3389/fimmu.2023.1129296. eCollection 2023. Front Immunol. 2023. PMID: 36923400 Free PMC article. Review.
-
Lipid nanoparticles for siRNA delivery in cancer treatment.J Control Release. 2023 Sep;361:130-146. doi: 10.1016/j.jconrel.2023.07.054. Epub 2023 Aug 4. J Control Release. 2023. PMID: 37532145 Review.
Cited by
-
Activation of Wnt/β-Catenin Signaling Involves 660 nm Laser Radiation on Epithelium and Modulates Lipid Metabolism.Biomolecules. 2022 Sep 29;12(10):1389. doi: 10.3390/biom12101389. Biomolecules. 2022. PMID: 36291598 Free PMC article.
-
A Novel Cuproptosis-Related Prognostic Model and the Hub Gene FDX1 Predict the Prognosis and Correlate with Immune Infiltration in Clear Cell Renal Cell Carcinoma.J Oncol. 2022 Dec 10;2022:2124088. doi: 10.1155/2022/2124088. eCollection 2022. J Oncol. 2022. PMID: 36536785 Free PMC article.
-
Tailoring combinatorial lipid nanoparticles for intracellular delivery of nucleic acids, proteins, and drugs.Acta Pharm Sin B. 2022 Jun;12(6):2624-2639. doi: 10.1016/j.apsb.2022.04.013. Epub 2022 Apr 27. Acta Pharm Sin B. 2022. PMID: 35755280 Free PMC article. Review.
-
Antitumor synergism between PAK4 silencing and immunogenic phototherapy of engineered extracellular vesicles.Acta Pharm Sin B. 2023 Sep;13(9):3945-3955. doi: 10.1016/j.apsb.2023.03.020. Epub 2023 Mar 29. Acta Pharm Sin B. 2023. PMID: 37719367 Free PMC article.
-
Acidic microenvironment responsive polymeric MOF-based nanoparticles induce immunogenic cell death for combined cancer therapy.J Nanobiotechnology. 2021 Dec 28;19(1):455. doi: 10.1186/s12951-021-01217-4. J Nanobiotechnology. 2021. PMID: 34963499 Free PMC article.
References
-
- (a) Akinc A., Maier M. A., Manoharan M., Fitzgerald K., Jayaraman M., Barros S., Ansell S., Du X., Hope M. J., Madden T. D., Mui B. L., Semple S. C., Tam Y. K., Ciufolini M., Witzigmann D., Kulkarni J. A., van der Meel R., Cullis P. R., Nat. Nanotechnol. 2019, 14, 1084; - PubMed
- (b) Balwani M., Sardh E., Ventura P., Peiró P. A., Rees D. C., Stölzel U., Bissell D. M., Bonkovsky H. L., Windyga J., Anderson K. E., Parker C., Silver S. M., Keel S. B., Wang J.‐D., Stein P. E., Harper P., Vassiliou D., Wang B., Phillips J., Ivanova A., Langendonk J. G., Kauppinen R., Minder E., Horie Y., Penz C., Chen J., Liu S., Ko J. J., Sweetser M. T., Garg P., Vaishnaw A., Kim J. B., Simon A. R., Gouya L., N. Engl. J. Med. 2020, 382, 2289; - PubMed
- (c) Lieberman J., Nat. Struct. Mol. Biol. 2018, 25, 357; - PMC - PubMed
- (d) Raal F. J., Kallend D., Ray K. A.‐O., Turner T., Koenig W., Wright R. S., Wijngaard P. L. J., Curcio D., Jaros M. J., Leiter L. A., Kastelein J. J. P., N. Engl. J. Med. 2020, 382, 1520. - PubMed
-
- Wang‐Gillam A., Li C.‐P., Bodoky G., Dean A., Shan Y.‐S., Jameson G., Macarulla T., Lee K.‐H., Cunningham D., Blanc J. F., Hubner R. A., Chiu C.‐F., Schwartsmann G., Siveke J. T., Braiteh F., Moyo V., Belanger B., Dhindsa N., Bayever E., Von Hoff D. D., Chen L.‐T., Adoo C., Anderson T., Asselah J., Azambuja A., Bampton C., Barrios C. H., Bekaii‐Saab T., Bohuslav M., Chang D., Chen J.‐S., Chen Y.‐C., Choi H. J., Chung I. J., Chung V., Csoszi T., Cubillo A., DeMarco L., de Wit M., Dragovich T., Edenfield W., Fein L. E., Franke F., Fuchs M., Gonzales‐Cruz V., Gozza A., Fernando R. H., Iaffaioli R., Jakesova J., Kahan Z., Karimi M., Kim J. S., Korbenfeld E., Lang I., Lee F.‐C., Lee K.‐D., Lipton L., Ma W. W., Mangel L., Mena R., Palmer D., Pant S., Park J. O., Piacentini P., Pelzer U., Plazas J. G., Prasad C., Rau K.‐M., Raoul J.‐L., Richards D., Ross P., Schlittler L., Smakal M., Stahalova V., Sternberg C., Seufferlein T., Tebbutt N., Vinholes J. J., Wadlow R., Wenczl M., Wong M., Lancet 2016, 387, 545. - PubMed
LinkOut - more resources
Full Text Sources
Miscellaneous