Using macular velocity measurements to relate parameters of bone conduction to vestibular compound action potential responses
- PMID: 37353559
- PMCID: PMC10290084
- DOI: 10.1038/s41598-023-37102-3
Using macular velocity measurements to relate parameters of bone conduction to vestibular compound action potential responses
Abstract
To examine mechanisms responsible for vestibular afferent sensitivity to transient bone conducted vibration, we performed simultaneous measurements of stimulus-evoked vestibular compound action potentials (vCAPs), utricular macula velocity, and vestibular microphonics (VMs) in anaesthetized guinea pigs. Results provide new insights into the kinematic variables of transient motion responsible for triggering mammalian vCAPs, revealing synchronized vestibular afferent responses are not universally sensitive to linear jerk as previously thought. For short duration stimuli (< 1 ms), the vCAP increases magnitude in close proportion to macular velocity and temporal bone (linear) acceleration, rather than other kinematic elements. For longer duration stimuli, the vCAP magnitude switches from temporal bone acceleration sensitive to linear jerk sensitive while maintaining macular velocity sensitivity. Frequency tuning curves evoked by tone-burst stimuli show vCAPs increase in proportion to onset macular velocity, while VMs increase in proportion to macular displacement across the entire frequency bandwidth tested between 0.1 and 2 kHz. The subset of vestibular afferent neurons responsible for synchronized firing and vCAPs have been shown previously to make calyceal synaptic contacts with type I hair cells in the striolar region of the epithelium and have irregularly spaced inter-spike intervals at rest. Present results provide new insight into mechanical and neural mechanisms underlying synchronized action potentials in these sensitive afferents, with clinical relevance for understanding the activation and tuning of neurons responsible for driving rapid compensatory reflex responses.
© 2023. The Author(s).
Conflict of interest statement
The authors declare no competing interests.
Figures
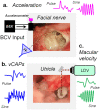
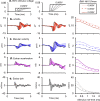
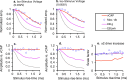
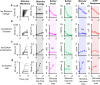
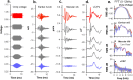
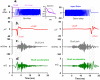
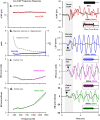
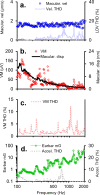
Similar articles
-
Evidence That Ultrafast Nonquantal Transmission Underlies Synchronized Vestibular Action Potential Generation.J Neurosci. 2023 Oct 25;43(43):7149-7157. doi: 10.1523/JNEUROSCI.1417-23.2023. Epub 2023 Sep 29. J Neurosci. 2023. PMID: 37775302 Free PMC article.
-
Bone conducted vibration selectively activates irregular primary otolithic vestibular neurons in the guinea pig.Exp Brain Res. 2006 Nov;175(2):256-67. doi: 10.1007/s00221-006-0544-1. Epub 2006 Jun 8. Exp Brain Res. 2006. PMID: 16761136
-
A mathematical model for mechanical activation and compound action potential generation by the utricle in response to sound and vibration.Front Neurol. 2023 Mar 27;14:1109506. doi: 10.3389/fneur.2023.1109506. eCollection 2023. Front Neurol. 2023. PMID: 37051057 Free PMC article.
-
Vestibular primary afferent responses to sound and vibration in the guinea pig.Exp Brain Res. 2011 May;210(3-4):347-52. doi: 10.1007/s00221-010-2499-5. Epub 2010 Nov 28. Exp Brain Res. 2011. PMID: 21113779 Review.
-
Neural basis of new clinical vestibular tests: otolithic neural responses to sound and vibration.Clin Exp Pharmacol Physiol. 2014 May;41(5):371-80. doi: 10.1111/1440-1681.12222. Clin Exp Pharmacol Physiol. 2014. PMID: 24754528 Review.
Cited by
-
Vestibular Testing-New Physiological Results for the Optimization of Clinical VEMP Stimuli.Audiol Res. 2023 Nov 9;13(6):910-928. doi: 10.3390/audiolres13060079. Audiol Res. 2023. PMID: 37987337 Free PMC article. Review.
-
Evidence That Ultrafast Nonquantal Transmission Underlies Synchronized Vestibular Action Potential Generation.J Neurosci. 2023 Oct 25;43(43):7149-7157. doi: 10.1523/JNEUROSCI.1417-23.2023. Epub 2023 Sep 29. J Neurosci. 2023. PMID: 37775302 Free PMC article.
References
Publication types
MeSH terms
Grants and funding
LinkOut - more resources
Full Text Sources