Dielectric metasurfaces for next-generation optical biosensing: a comparison with plasmonic sensing
- PMID: 37352839
- PMCID: PMC10416613
- DOI: 10.1088/1361-6528/ace117
Dielectric metasurfaces for next-generation optical biosensing: a comparison with plasmonic sensing
Abstract
In the past decades, nanophotonic biosensors have been extended from the extensively studied plasmonic platforms to dielectric metasurfaces. Instead of plasmonic resonance, dielectric metasurfaces are based on Mie resonance, and provide comparable sensitivity with superior resonance bandwidth, Q factor, and figure-of-merit. Although the plasmonic photothermal effect is beneficial in many biomedical applications, it is a fundamental limitation for biosensing. Dielectric metasurfaces solve the ohmic loss and heating problems, providing better repeatability, stability, and biocompatibility. We review the high-Q resonances based on various physical phenomena tailored by meta-atom geometric designs, and compare dielectric and plasmonic metasurfaces in refractometric, surface-enhanced, and chiral sensing for various biomedical and diagnostic applications. Departing from conventional spectral shift measurement using spectrometers, imaging-based and spectrometer-less biosensing are highlighted, including single-wavelength refractometric barcoding, surface-enhanced molecular fingerprinting, and integrated visual reporting. These unique modalities enabled by dielectric metasurfaces point to two important research directions. On the one hand, hyperspectral imaging provides massive information for smart data processing, which not only achieve better biomolecular sensing performance than conventional ensemble averaging, but also enable real-time monitoring of cellular or microbial behaviour in physiological conditions. On the other hand, a single metasurface can integrate both functions of sensing and optical output engineering, using single-wavelength or broadband light sources, which provides simple, fast, compact, and cost-effective solutions. Finally, we provide perspectives in future development on metasurface nanofabrication, functionalization, material, configuration, and integration, towards next-generation optical biosensing for ultra-sensitive, portable/wearable, lab-on-a-chip, point-of-care, multiplexed, and scalable applications.
Keywords: Mie resonance; biosensing; bound states in the continuum; dielectric metasurface; nanophotonics; plasmonics; point-of-care.
© 2023 IOP Publishing Ltd.
Figures
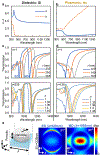
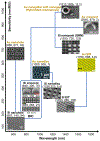
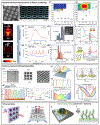
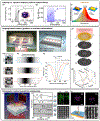
Similar articles
-
Exploring near-field sensing efficiency of complementary plasmonic metasurfaces for immunodetection of tumor markers.Biosens Bioelectron. 2022 May 1;203:114038. doi: 10.1016/j.bios.2022.114038. Epub 2022 Jan 26. Biosens Bioelectron. 2022. PMID: 35121450
-
Gradient High-Q Dielectric Metasurfaces for Broadband Sensing and Control of Vibrational Light-Matter Coupling.Adv Mater. 2024 Jun;36(25):e2314279. doi: 10.1002/adma.202314279. Epub 2024 Apr 9. Adv Mater. 2024. PMID: 38511549
-
Bound States in the Continuum in Anisotropic Plasmonic Metasurfaces.Nano Lett. 2020 Sep 9;20(9):6351-6356. doi: 10.1021/acs.nanolett.0c01752. Epub 2020 Jun 10. Nano Lett. 2020. PMID: 32479094
-
Metasurface-Based Molecular Biosensing Aided by Artificial Intelligence.Angew Chem Int Ed Engl. 2019 Oct 14;58(42):14810-14822. doi: 10.1002/anie.201901443. Epub 2019 Aug 8. Angew Chem Int Ed Engl. 2019. PMID: 31021045 Review.
-
Nonlinear Optics in Dielectric Guided-Mode Resonant Structures and Resonant Metasurfaces.Micromachines (Basel). 2020 Apr 24;11(4):449. doi: 10.3390/mi11040449. Micromachines (Basel). 2020. PMID: 32344556 Free PMC article. Review.
Cited by
-
All-Dielectric Metasurface-Based Terahertz Molecular Fingerprint Sensor for Trace Cinnamoylglycine Detection.Biosensors (Basel). 2024 Sep 13;14(9):440. doi: 10.3390/bios14090440. Biosensors (Basel). 2024. PMID: 39329815 Free PMC article.
-
Terahertz Biosensor Engineering Based on Quasi-BIC Metasurface with Ultrasensitive Detection.Nanomaterials (Basel). 2024 May 4;14(9):799. doi: 10.3390/nano14090799. Nanomaterials (Basel). 2024. PMID: 38727393 Free PMC article.
-
TiO2 Nano-Biopatterning Reveals Optimal Ligand Presentation for Cell-Matrix Adhesion Formation.Adv Mater. 2024 May;36(21):e2309284. doi: 10.1002/adma.202309284. Epub 2024 Feb 19. Adv Mater. 2024. PMID: 38340044
References
-
- McDonagh C, Burke CS and MacCraith BD 2008. Optical chemical sensors Chem Rev 108 400–22 - PubMed
-
- Altug H, Oh S-H, Maier SA and Homola J 2022. Advances and applications of nanophotonic biosensors Nat Nanotechnol 17 5–16 - PubMed
-
- Anker JN, Hall WP, Lyandres O, Shah NC, Zhao J and Van Duyne R P 2008. Biosensing with plasmonic nanosensors Nat Mater 7 442–53 - PubMed
-
- Schuller JA, Barnard ES, Cai WS, Jun YC, White JS and Brongersma ML 2010. Plasmonics for extreme light concentration and manipulation Nat Mater 9 193–204 - PubMed
Publication types
MeSH terms
Grants and funding
LinkOut - more resources
Full Text Sources
Miscellaneous