Defining diurnal fluctuations in mouse choroid plexus and CSF at high molecular, spatial, and temporal resolution
- PMID: 37349305
- PMCID: PMC10287727
- DOI: 10.1038/s41467-023-39326-3
Defining diurnal fluctuations in mouse choroid plexus and CSF at high molecular, spatial, and temporal resolution
Abstract
Transmission and secretion of signals via the choroid plexus (ChP) brain barrier can modulate brain states via regulation of cerebrospinal fluid (CSF) composition. Here, we developed a platform to analyze diurnal variations in male mouse ChP and CSF. Ribosome profiling of ChP epithelial cells revealed diurnal translatome differences in metabolic machinery, secreted proteins, and barrier components. Using ChP and CSF metabolomics and blood-CSF barrier analyses, we observed diurnal changes in metabolites and cellular junctions. We then focused on transthyretin (TTR), a diurnally regulated thyroid hormone chaperone secreted by the ChP. Diurnal variation in ChP TTR depended on Bmal1 clock gene expression. We achieved real-time tracking of CSF-TTR in awake TtrmNeonGreen mice via multi-day intracerebroventricular fiber photometry. Diurnal changes in ChP and CSF TTR levels correlated with CSF thyroid hormone levels. These datasets highlight an integrated platform for investigating diurnal control of brain states by the ChP and CSF.
© 2023. The Author(s).
Conflict of interest statement
The authors declare no competing interests.
Figures
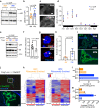
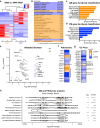
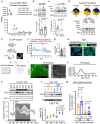
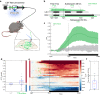
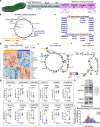
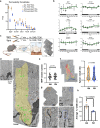
Similar articles
-
Role of transthyretin in the transport of thyroxine from the blood to the choroid plexus, the cerebrospinal fluid, and the brain.Endocrinology. 1992 Feb;130(2):933-8. doi: 10.1210/endo.130.2.1733735. Endocrinology. 1992. PMID: 1733735
-
Transthyretin regulates thyroid hormone levels in the choroid plexus, but not in the brain parenchyma: study in a transthyretin-null mouse model.Endocrinology. 2000 Sep;141(9):3267-72. doi: 10.1210/endo.141.9.7659. Endocrinology. 2000. PMID: 10965897
-
Inhibition by lead of production and secretion of transthyretin in the choroid plexus: its relation to thyroxine transport at blood-CSF barrier.Toxicol Appl Pharmacol. 1999 Feb 15;155(1):24-31. doi: 10.1006/taap.1998.8611. Toxicol Appl Pharmacol. 1999. PMID: 10036215 Free PMC article.
-
The role of transthyretin in the transport of thyroid hormone to cerebrospinal fluid and brain.Acta Med Austriaca. 1992;19 Suppl 1:25-8. Acta Med Austriaca. 1992. PMID: 1519447 Review.
-
The evolutionary and integrative roles of transthyretin in thyroid hormone homeostasis.J Endocrinol. 2002 Oct;175(1):61-73. doi: 10.1677/joe.0.1750061. J Endocrinol. 2002. PMID: 12379491 Review.
Cited by
-
Optimized Mass Spectrometry Detection of Thyroid Hormones and Polar Metabolites in Rodent Cerebrospinal Fluid.Metabolites. 2024 Jan 23;14(2):79. doi: 10.3390/metabo14020079. Metabolites. 2024. PMID: 38392972 Free PMC article.
-
Day-night fluctuations in choroid plexus transcriptomics and cerebrospinal fluid metabolomics.PNAS Nexus. 2023 Aug 10;2(8):pgad262. doi: 10.1093/pnasnexus/pgad262. eCollection 2023 Aug. PNAS Nexus. 2023. PMID: 37614671 Free PMC article.
-
c-fos induction in the choroid plexus, tanycytes and pars tuberalis is an early indicator of spontaneous arousal from torpor in a deep hibernator.J Exp Biol. 2024 May 15;227(10):jeb247224. doi: 10.1242/jeb.247224. Epub 2024 May 23. J Exp Biol. 2024. PMID: 38690647 Free PMC article.
-
Multiciliated ependymal cells: an update on biology and pathology in the adult brain.Acta Neuropathol. 2024 Sep 10;148(1):39. doi: 10.1007/s00401-024-02784-0. Acta Neuropathol. 2024. PMID: 39254862 Review.
-
Breakthroughs in choroid plexus and CSF biology from the first European Choroid plexus Scientific Forum (ECSF).Fluids Barriers CNS. 2024 May 21;21(1):43. doi: 10.1186/s12987-024-00546-4. Fluids Barriers CNS. 2024. PMID: 38773599 Free PMC article. Review.
References
-
- Nilsson C, et al. Circadian variation in human cerebrospinal fluid production measured by magnetic resonance imaging. Am. J. Physiol. 1992;262:R20–R24. - PubMed
Publication types
MeSH terms
Substances
Grants and funding
- R01 NS088566/NS/NINDS NIH HHS/United States
- P50 HD105351/HD/NICHD NIH HHS/United States
- T32 HL007901/HL/NHLBI NIH HHS/United States
- R01 HL151368/HL/NHLBI NIH HHS/United States
- OT2 OD030544/OD/NIH HHS/United States
- T32 GM144273/GM/NIGMS NIH HHS/United States
- T32 GM008313/GM/NIGMS NIH HHS/United States
- R35 HL145242/HL/NHLBI NIH HHS/United States
- RF1 DA048790/DA/NIDA NIH HHS/United States
- R01 AI130591/AI/NIAID NIH HHS/United States
- U2C DK119886/DK/NIDDK NIH HHS/United States
- T32 GM007753/GM/NIGMS NIH HHS/United States
- T32 HL110852/HL/NHLBI NIH HHS/United States
- F30 DK131642/DK/NIDDK NIH HHS/United States
- U54 HD090255/HD/NICHD NIH HHS/United States
LinkOut - more resources
Full Text Sources
Molecular Biology Databases
Research Materials
Miscellaneous