Genotoxic aldehyde stress prematurely ages hematopoietic stem cells in a p53-driven manner
- PMID: 37348497
- PMCID: PMC7614878
- DOI: 10.1016/j.molcel.2023.05.035
Genotoxic aldehyde stress prematurely ages hematopoietic stem cells in a p53-driven manner
Abstract
Aged hematopoietic stem cells (HSCs) display diminished self-renewal and a myeloid differentiation bias. However, the drivers and mechanisms that underpin this fundamental switch are not understood. HSCs produce genotoxic formaldehyde that requires protection by the detoxification enzymes ALDH2 and ADH5 and the Fanconi anemia (FA) DNA repair pathway. We find that the HSCs in young Aldh2-/-Fancd2-/- mice harbor a transcriptomic signature equivalent to aged wild-type HSCs, along with increased epigenetic age, telomere attrition, and myeloid-biased differentiation quantified by single HSC transplantation. In addition, the p53 response is vigorously activated in Aldh2-/-Fancd2-/- HSCs, while p53 deletion rescued this aged HSC phenotype. To further define the origins of the myeloid differentiation bias, we use a GFP genetic reporter to find a striking enrichment of Vwf+ myeloid and megakaryocyte-lineage-biased HSCs. These results indicate that metabolism-derived formaldehyde-DNA damage stimulates the p53 response in HSCs to drive accelerated aging.
Keywords: DNA damage; DNA-damage response; aging; aldehydes; hematopoiesis; myeloid bias; p53.
Copyright © 2023 The Authors. Published by Elsevier Inc. All rights reserved.
Conflict of interest statement
Declaration of interests The authors declare no competing interests.
Figures
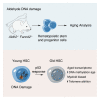
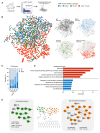
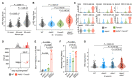
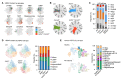
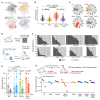
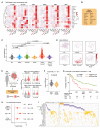
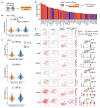
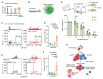
Similar articles
-
Genotoxic consequences of endogenous aldehydes on mouse haematopoietic stem cell function.Nature. 2012 Sep 27;489(7417):571-5. doi: 10.1038/nature11368. Epub 2012 Aug 26. Nature. 2012. PMID: 22922648
-
Organelle dysfunction upon asrij depletion causes aging-like changes in mouse hematopoietic stem cells.Aging Cell. 2022 Apr;21(4):e13570. doi: 10.1111/acel.13570. Epub 2022 Mar 15. Aging Cell. 2022. PMID: 35289070 Free PMC article.
-
p53-TP53-Induced Glycolysis Regulator Mediated Glycolytic Suppression Attenuates DNA Damage and Genomic Instability in Fanconi Anemia Hematopoietic Stem Cells.Stem Cells. 2019 Jul;37(7):937-947. doi: 10.1002/stem.3015. Epub 2019 May 3. Stem Cells. 2019. PMID: 30977208 Free PMC article.
-
DNA damage and repair in the hematopoietic system.Acta Biochim Biophys Sin (Shanghai). 2022 Jan 25;54(6):847-857. doi: 10.3724/abbs.2022053. Acta Biochim Biophys Sin (Shanghai). 2022. PMID: 35593466 Free PMC article. Review.
-
Protection of hematopoietic stem cells from stress-induced exhaustion and aging.Curr Opin Hematol. 2020 Jul;27(4):225-231. doi: 10.1097/MOH.0000000000000586. Curr Opin Hematol. 2020. PMID: 32398455 Review.
Cited by
-
Ageing-related bone and immunity changes: insights into the complex interplay between the skeleton and the immune system.Bone Res. 2024 Aug 5;12(1):42. doi: 10.1038/s41413-024-00346-4. Bone Res. 2024. PMID: 39103328 Free PMC article. Review.
-
The DNA helicase FANCJ (BRIP1) functions in double strand break repair processing, but not crossover formation during prophase I of meiosis in male mice.PLoS Genet. 2024 Feb 20;20(2):e1011175. doi: 10.1371/journal.pgen.1011175. eCollection 2024 Feb. PLoS Genet. 2024. PMID: 38377115 Free PMC article.
-
A cellular identity crisis? Plasticity changes during aging and rejuvenation.Genes Dev. 2024 Oct 16;38(17-20):823-842. doi: 10.1101/gad.351728.124. Genes Dev. 2024. PMID: 39293862 Review.
-
Friend or foe-maldehyde.Nat Chem Biol. 2024 Mar;20(3):268-270. doi: 10.1038/s41589-024-01558-9. Nat Chem Biol. 2024. PMID: 38424170 No abstract available.
-
ALDH1A3-acetaldehyde metabolism potentiates transcriptional heterogeneity in melanoma.Cell Rep. 2024 Jul 23;43(7):114406. doi: 10.1016/j.celrep.2024.114406. Epub 2024 Jul 3. Cell Rep. 2024. PMID: 38963759 Free PMC article.
References
Publication types
MeSH terms
Substances
Grants and funding
- MC_PC_17230/MRC_/Medical Research Council/United Kingdom
- C1163/A21762/CRUK_/Cancer Research UK/United Kingdom
- MC_UU_00029/1/MRC_/Medical Research Council/United Kingdom
- 18002/LLR_/Blood Cancer UK/United Kingdom
- 23015/CRUK_/Cancer Research UK/United Kingdom
- MR/S036113/1/MRC_/Medical Research Council/United Kingdom
- 21762/CRUK_/Cancer Research UK/United Kingdom
- C42693/A23273/CRUK_/Cancer Research UK/United Kingdom
- 203151/WT_/Wellcome Trust/United Kingdom
- C60150/A23919/CRUK_/Cancer Research UK/United Kingdom
- 106202/Z/14/Z/WT_/Wellcome Trust/United Kingdom
- 203151/Z/16/Z/WT_/Wellcome Trust/United Kingdom
- 206328/Z/17/Z/WT_/Wellcome Trust/United Kingdom
- MC_UU_00016/17/MRC_/Medical Research Council/United Kingdom
- MC_U105178811/MRC_/Medical Research Council/United Kingdom
- WT_/Wellcome Trust/United Kingdom
LinkOut - more resources
Full Text Sources
Medical
Molecular Biology Databases
Research Materials
Miscellaneous