Hindbrain modules differentially transform activity of single collicular neurons to coordinate movements
- PMID: 37343561
- PMCID: PMC10424787
- DOI: 10.1016/j.cell.2023.05.031
Hindbrain modules differentially transform activity of single collicular neurons to coordinate movements
Abstract
Seemingly simple behaviors such as swatting a mosquito or glancing at a signpost involve the precise coordination of multiple body parts. Neural control of coordinated movements is widely thought to entail transforming a desired overall displacement into displacements for each body part. Here we reveal a different logic implemented in the mouse gaze system. Stimulating superior colliculus (SC) elicits head movements with stereotyped displacements but eye movements with stereotyped endpoints. This is achieved by individual SC neurons whose branched axons innervate modules in medulla and pons that drive head movements with stereotyped displacements and eye movements with stereotyped endpoints, respectively. Thus, single neurons specify a mixture of endpoints and displacements for different body parts, not overall displacement, with displacements for different body parts computed at distinct anatomical stages. Our study establishes an approach for unraveling motor hierarchies and identifies a logic for coordinating movements and the resulting pose.
Keywords: ethology; gaze; hindbrain; motor control; movement coordination; orienting; saccades; signal multiplexing; superior colliculus; systems neuroscience; upper motor neurons.
Copyright © 2023 Elsevier Inc. All rights reserved.
Conflict of interest statement
Declaration of interests The authors declare no competing interests.
Figures
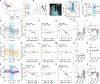
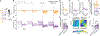
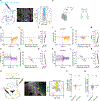
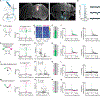
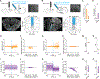
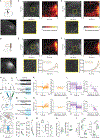
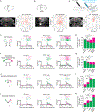
Similar articles
-
Brain stem omnipause neurons and the control of combined eye-head gaze saccades in the alert cat.J Neurophysiol. 1998 Jun;79(6):3060-76. doi: 10.1152/jn.1998.79.6.3060. J Neurophysiol. 1998. PMID: 9636108
-
Activity of cells in the deeper layers of the superior colliculus of the rhesus monkey: evidence for a gaze displacement command.J Neurophysiol. 1997 Sep;78(3):1669-90. doi: 10.1152/jn.1997.78.3.1669. J Neurophysiol. 1997. PMID: 9310452
-
Gaze shifts evoked by stimulation of the superior colliculus in the head-free cat conform to the motor map but also depend on stimulus strength and fixation activity.Exp Brain Res. 1994;101(1):123-39. doi: 10.1007/BF00243222. Exp Brain Res. 1994. PMID: 7843291
-
Translation of sensory signals into commands for control of saccadic eye movements: role of primate superior colliculus.Physiol Rev. 1986 Jan;66(1):118-71. doi: 10.1152/physrev.1986.66.1.118. Physiol Rev. 1986. PMID: 3511480 Review.
-
Neural control of 3-D gaze shifts in the primate.Prog Brain Res. 2003;142:109-24. doi: 10.1016/s0079-6123(03)42009-8. Prog Brain Res. 2003. PMID: 12693257 Review.
Cited by
-
Basal ganglia-spinal cord pathway that commands locomotor gait asymmetries in mice.Nat Neurosci. 2024 Apr;27(4):716-727. doi: 10.1038/s41593-024-01569-8. Epub 2024 Feb 12. Nat Neurosci. 2024. PMID: 38347200 Free PMC article.
-
Photoacoustic: A Versatile Nongenetic Method for High-Precision Neuromodulation.Acc Chem Res. 2024 Jun 4;57(11):1595-1607. doi: 10.1021/acs.accounts.4c00119. Epub 2024 May 17. Acc Chem Res. 2024. PMID: 38759211 Free PMC article. Review.
-
Genetically defined neuron types underlying visuomotor transformation in the superior colliculus.Nat Rev Neurosci. 2024 Nov;25(11):726-739. doi: 10.1038/s41583-024-00856-4. Epub 2024 Sep 27. Nat Rev Neurosci. 2024. PMID: 39333418 Review.
References
-
- Kandel ER, Schwartz JH, Jessell TM, Siegelbaum SA & Hudspeth AJ. (2013) Principles of Neural Science, Fifth Editon | Neurology Collection | McGraw-Hill Medical. 2013.