Exploring skeletal muscle tolerance and whole-body metabolic effects of FDA-approved drugs in a volumetric muscle loss model
- PMID: 37332022
- PMCID: PMC10277213
- DOI: 10.14814/phy2.15756
Exploring skeletal muscle tolerance and whole-body metabolic effects of FDA-approved drugs in a volumetric muscle loss model
Abstract
Volumetric muscle loss (VML) is associated with persistent functional impairment due to a lack of de novo muscle regeneration. As mechanisms driving the lack of regeneration continue to be established, adjunctive pharmaceuticals to address the pathophysiology of the remaining muscle may offer partial remediation. Studies were designed to evaluate the tolerance and efficacy of two FDA-approved pharmaceutical modalities to address the pathophysiology of the remaining muscle tissue after VML injury: (1) nintedanib (an anti-fibrotic) and (2) combined formoterol and leucine (myogenic promoters). Tolerance was first established by testing low- and high-dosage effects on uninjured skeletal muscle mass and myofiber cross-sectional area in adult male C57BL/6J mice. Next, tolerated doses of the two pharmaceutical modalities were tested in VML-injured adult male C57BL/6J mice after an 8-week treatment period for their ability to modulate muscle strength and whole-body metabolism. The most salient findings indicate that formoterol plus leucine mitigated the loss in muscle mass, myofiber number, whole-body lipid oxidation, and muscle strength, and resulted in a higher whole-body metabolic rate (p ≤ 0.016); nintedanib did not exacerbate or correct aspects of the muscle pathophysiology after VML. This supports ongoing optimization efforts, including scale-up evaluations of formoterol treatment in large animal models of VML.
Keywords: formoterol; muscle function; neuromusculoskeletal injury; nintedanib; skeletal muscle injury.
© 2023 The Authors. Physiological Reports published by Wiley Periodicals LLC on behalf of The Physiological Society and the American Physiological Society.
Conflict of interest statement
The authors declare that they have no conflict of interests.
Figures
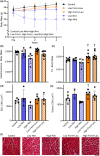
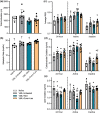
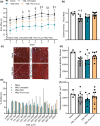
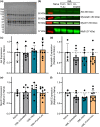
Similar articles
-
Restricted physical activity after volumetric muscle loss alters whole-body and local muscle metabolism.J Physiol. 2023 Feb;601(4):743-761. doi: 10.1113/JP283959. Epub 2023 Jan 3. J Physiol. 2023. PMID: 36536512 Free PMC article.
-
Pharmaceutical Agents for Contractile-Metabolic Dysfunction After Volumetric Muscle Loss.Tissue Eng Part A. 2022 Sep;28(17-18):795-806. doi: 10.1089/ten.TEA.2022.0036. Epub 2022 Aug 1. Tissue Eng Part A. 2022. PMID: 35620911 Free PMC article.
-
Resistance wheel running improves contractile strength, but not metabolic capacity, in a murine model of volumetric muscle loss injury.Exp Physiol. 2023 Oct;108(10):1282-1294. doi: 10.1113/EP091284. Epub 2023 Aug 10. Exp Physiol. 2023. PMID: 37526646 Free PMC article.
-
Pathophysiology of Volumetric Muscle Loss Injury.Cells Tissues Organs. 2016;202(3-4):180-188. doi: 10.1159/000443925. Epub 2016 Nov 9. Cells Tissues Organs. 2016. PMID: 27825160 Review.
-
Immunomodulation and Biomaterials: Key Players to Repair Volumetric Muscle Loss.Cells. 2021 Aug 7;10(8):2016. doi: 10.3390/cells10082016. Cells. 2021. PMID: 34440785 Free PMC article. Review.
References
-
- Anthony, J. C. , Yoshizawa, F. , Anthony, T. G. , Vary, T. C. , Jefferson, L. S. , & Kimball, S. R. (2000). Leucine stimulates translation initiation in skeletal muscle of postabsorptive rats via a rapamycin‐sensitive pathway. The Journal of Nutrition, 130, 2413–2419. - PubMed
-
- Bedair, H. S. , Karthikeyan, T. , Quintero, A. , Li, Y. , & Huard, J. (2008). Angiotensin II receptor blockade administered after injury improves muscle regeneration and decreases fibrosis in normal skeletal muscle. The American Journal of Sports Medicine, 36, 1548–1554. - PubMed
-
- Buchholz, A. C. , Rafii, M. , & Pencharz, P. B. (2001). Is resting metabolic rate different between men and women? The British Journal of Nutrition, 86, 641–646. - PubMed
Publication types
MeSH terms
Substances
LinkOut - more resources
Full Text Sources
Medical
Research Materials