Vital for Viruses: Intrinsically Disordered Proteins
- PMID: 37330280
- PMCID: PMC10656058
- DOI: 10.1016/j.jmb.2022.167860
Vital for Viruses: Intrinsically Disordered Proteins
Abstract
Viruses infect all kingdoms of life; their genomes vary from DNA to RNA and in size from 2kB to 1 MB or more. Viruses frequently employ disordered proteins, that is, protein products of virus genes that do not themselves fold into independent three-dimensional structures, but rather, constitute a versatile molecular toolkit to accomplish a range of functions necessary for viral infection, assembly, and proliferation. Interestingly, disordered proteins have been discovered in almost all viruses so far studied, whether the viral genome consists of DNA or RNA, and whatever the configuration of the viral capsid or other outer covering. In this review, I present a wide-ranging set of stories illustrating the range of functions of IDPs in viruses. The field is rapidly expanding, and I have not tried to include everything. What is included is meant to be a survey of the variety of tasks that viruses accomplish using disordered proteins.
Keywords: Protein disorder; post-translational modification; protein–protein interaction; viral oncoproteins; virus proteins.
Copyright © 2022 Elsevier Ltd. All rights reserved.
Conflict of interest statement
The author declares no conflict of interest
Figures
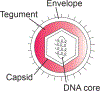

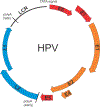

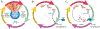
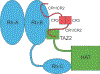
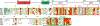
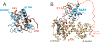

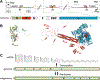
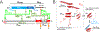
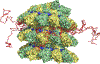
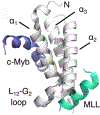
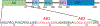
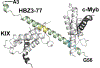
Similar articles
-
Nucleic acid packaging in viruses.Curr Opin Struct Biol. 2012 Feb;22(1):65-71. doi: 10.1016/j.sbi.2011.11.002. Epub 2012 Jan 23. Curr Opin Struct Biol. 2012. PMID: 22277169 Free PMC article. Review.
-
Multiple layers of chimerism in a single-stranded DNA virus discovered by deep sequencing.Genome Biol Evol. 2015 Apr 2;7(4):993-1001. doi: 10.1093/gbe/evv034. Genome Biol Evol. 2015. PMID: 25840414 Free PMC article.
-
Viral genome organization.Adv Protein Chem. 2003;64:219-58. doi: 10.1016/s0065-3233(03)01006-4. Adv Protein Chem. 2003. PMID: 13677049 Review. No abstract available.
-
Deep Recombination: RNA and ssDNA Virus Genes in DNA Virus and Host Genomes.Annu Rev Virol. 2015 Nov;2(1):203-17. doi: 10.1146/annurev-virology-100114-055127. Epub 2015 Sep 2. Annu Rev Virol. 2015. PMID: 26958913 Review.
-
What does structure tell us about virus evolution?Curr Opin Struct Biol. 2005 Dec;15(6):655-63. doi: 10.1016/j.sbi.2005.10.012. Epub 2005 Nov 3. Curr Opin Struct Biol. 2005. PMID: 16271469 Review.
Cited by
-
Diversity and Complexity of Internally Deleted Viral Genomes in Influenza A Virus Subpopulations with Enhanced Interferon-Inducing Phenotypes.Viruses. 2023 Oct 17;15(10):2107. doi: 10.3390/v15102107. Viruses. 2023. PMID: 37896883 Free PMC article.
-
Structural Model of Bacteriophage P22 Scaffolding Protein in a Procapsid by Magic-Angle Spinning NMR.bioRxiv [Preprint]. 2024 Nov 1:2024.11.01.621488. doi: 10.1101/2024.11.01.621488. bioRxiv. 2024. PMID: 39554170 Free PMC article. Preprint.
-
Conserved molecular recognition by an intrinsically disordered region in the absence of sequence conservation.Res Sq [Preprint]. 2024 Jun 3:rs.3.rs-4477977. doi: 10.21203/rs.3.rs-4477977/v1. Res Sq. 2024. PMID: 38883712 Free PMC article. Preprint.
-
Molecular simulations integrated with experiments for probing the interaction dynamics and binding mechanisms of intrinsically disordered proteins.Curr Opin Struct Biol. 2024 Feb;84:102756. doi: 10.1016/j.sbi.2023.102756. Epub 2023 Dec 19. Curr Opin Struct Biol. 2024. PMID: 38118365 Free PMC article. Review.
References
-
- Wright PE, Dyson HJ, (1999). Intrinsically unstructured proteins: Re-assessing the protein structure-function paradigm. J. Mol. Biol, 293, 321–331. - PubMed
-
- Piersimoni L, Abd el Malek M, Bhatia T, Bender J, Brankatschk C, Calvo Sánchez J, Dayhoff GW, Di Ianni A, Figueroa Parra JO, Garcia-Martinez D, Hesselbarth J, Köppen J, Lauth LM, Lippik L, Machner L, Sachan S, Schmidt L, Selle R, Skalidis I, Sorokin O, Ubbiali D, Voigt B, Wedler A, Wei AAJ, Zorn P, Dunker AK, Köhn M, Sinz A, Uversky VN, (2022). Lighting up Nobel Prize-winning studies with protein intrinsic disorder. Cell. Mol. Life Sci, 79, 449. - PMC - PubMed
-
- Iakoucheva LM, Brown CJ, Lawson JD, Obradovic Z, Dunker AK, (2002). Intrinsic disorder in cell-signaling and cancer-associated proteins. J. Mol. Biol, 323, 573–584. - PubMed
-
- Davey NE, Travé G, Gibson TJ, (2011). How viruses hijack cell regulation. Trends Biochem. Sci, 36, 159–169. - PubMed
Publication types
MeSH terms
Substances
Grants and funding
LinkOut - more resources
Full Text Sources
Miscellaneous