Optimized testing strategy for the diagnosis of GAA-FGF14 ataxia/spinocerebellar ataxia 27B
- PMID: 37322040
- PMCID: PMC10272173
- DOI: 10.1038/s41598-023-36654-8
Optimized testing strategy for the diagnosis of GAA-FGF14 ataxia/spinocerebellar ataxia 27B
Abstract
Dominantly inherited GAA repeat expansions in FGF14 are a common cause of spinocerebellar ataxia (GAA-FGF14 ataxia; spinocerebellar ataxia 27B). Molecular confirmation of FGF14 GAA repeat expansions has thus far mostly relied on long-read sequencing, a technology that is not yet widely available in clinical laboratories. We developed and validated a strategy to detect FGF14 GAA repeat expansions using long-range PCR, bidirectional repeat-primed PCRs, and Sanger sequencing. We compared this strategy to targeted nanopore sequencing in a cohort of 22 French Canadian patients and next validated it in a cohort of 53 French index patients with unsolved ataxia. Method comparison showed that capillary electrophoresis of long-range PCR amplification products significantly underestimated expansion sizes compared to nanopore sequencing (slope, 0.87 [95% CI, 0.81 to 0.93]; intercept, 14.58 [95% CI, - 2.48 to 31.12]) and gel electrophoresis (slope, 0.84 [95% CI, 0.78 to 0.97]; intercept, 21.34 [95% CI, - 27.66 to 40.22]). The latter techniques yielded similar size estimates. Following calibration with internal controls, expansion size estimates were similar between capillary electrophoresis and nanopore sequencing (slope: 0.98 [95% CI, 0.92 to 1.04]; intercept: 10.62 [95% CI, - 7.49 to 27.71]), and gel electrophoresis (slope: 0.94 [95% CI, 0.88 to 1.09]; intercept: 18.81 [95% CI, - 41.93 to 39.15]). Diagnosis was accurately confirmed for all 22 French Canadian patients using this strategy. We also identified 9 French patients (9/53; 17%) and 2 of their relatives who carried an FGF14 (GAA)≥250 expansion. This novel strategy reliably detected and sized FGF14 GAA expansions, and compared favorably to long-read sequencing.
© 2023. The Author(s).
Conflict of interest statement
Matthis Synofzik has received consultancy honoraria from Janssen, Ionis, Orphazyme, Servier, Reata, GenOrph, and AviadoBio, all unrelated to the present manuscript. Stephan Zuchner is consultant on drug targets for Aeglea BioTherapeutics and consultant on clinical trial design for Applied Therapeutics, all of them unrelated to the work in the present manuscript. Other authors have no relevant financial interests to disclose.
Figures
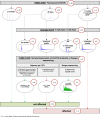
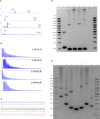
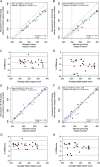
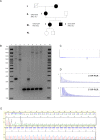
Similar articles
-
The genetic landscape and phenotypic spectrum of GAA-FGF14 ataxia in China: a large cohort study.EBioMedicine. 2024 Apr;102:105077. doi: 10.1016/j.ebiom.2024.105077. Epub 2024 Mar 20. EBioMedicine. 2024. PMID: 38513302 Free PMC article.
-
The FGF14 GAA repeat expansion in Greek patients with late-onset cerebellar ataxia and an overview of the SCA27B phenotype across populations.Clin Genet. 2024 Apr;105(4):446-452. doi: 10.1111/cge.14482. Epub 2024 Jan 14. Clin Genet. 2024. PMID: 38221848
-
Deep Intronic FGF14 GAA Repeat Expansion in Late-Onset Cerebellar Ataxia.N Engl J Med. 2023 Jan 12;388(2):128-141. doi: 10.1056/NEJMoa2207406. Epub 2022 Dec 14. N Engl J Med. 2023. PMID: 36516086 Free PMC article.
-
Spinocerebellar ataxia 27B: A novel, frequent and potentially treatable ataxia.Clin Transl Med. 2024 Jan;14(1):e1504. doi: 10.1002/ctm2.1504. Clin Transl Med. 2024. PMID: 38279833 Free PMC article. Review.
-
An Update on the Adult-Onset Hereditary Cerebellar Ataxias: Novel Genetic Causes and New Diagnostic Approaches.Cerebellum. 2024 Oct;23(5):2152-2168. doi: 10.1007/s12311-024-01703-z. Epub 2024 May 18. Cerebellum. 2024. PMID: 38760634 Free PMC article. Review.
Cited by
-
A common flanking variant is associated with enhanced stability of the FGF14-SCA27B repeat locus.Nat Genet. 2024 Jul;56(7):1366-1370. doi: 10.1038/s41588-024-01808-5. Epub 2024 Jun 27. Nat Genet. 2024. PMID: 38937606 Free PMC article.
-
Clinical and genetic keys to cerebellar ataxia due to FGF14 GAA expansions.EBioMedicine. 2024 Jan;99:104931. doi: 10.1016/j.ebiom.2023.104931. Epub 2023 Dec 27. EBioMedicine. 2024. PMID: 38150853 Free PMC article.
-
The genetic landscape and phenotypic spectrum of GAA-FGF14 ataxia in China: a large cohort study.EBioMedicine. 2024 Apr;102:105077. doi: 10.1016/j.ebiom.2024.105077. Epub 2024 Mar 20. EBioMedicine. 2024. PMID: 38513302 Free PMC article.
-
4-Aminopyridine improves real-life gait performance in SCA27B on a single-subject level: a prospective n-of-1 treatment experience.J Neurol. 2023 Nov;270(11):5629-5634. doi: 10.1007/s00415-023-11868-y. Epub 2023 Jul 13. J Neurol. 2023. PMID: 37439944 Free PMC article. No abstract available.
-
Hereditary Ataxias: From Bench to Clinic, Where Do We Stand?Cells. 2024 Feb 9;13(4):319. doi: 10.3390/cells13040319. Cells. 2024. PMID: 38391932 Free PMC article. Review.
References
Publication types
MeSH terms
Substances
Grants and funding
LinkOut - more resources
Full Text Sources
Medical
Miscellaneous