Human umbilical cord mesenchymal stem cell-derived exosomes ameliorate liver steatosis by promoting fatty acid oxidation and reducing fatty acid synthesis
- PMID: 37274776
- PMCID: PMC10232730
- DOI: 10.1016/j.jhepr.2023.100746
Human umbilical cord mesenchymal stem cell-derived exosomes ameliorate liver steatosis by promoting fatty acid oxidation and reducing fatty acid synthesis
Abstract
Background & aims: Non-alcoholic fatty liver disease (NAFLD) affects nearly a quarter of the population with no approved pharmacological therapy. Liver steatosis is a primary characteristic of NAFLD. Recent studies suggest that human umbilical cord mesenchymal stem cell-derived exosomes (MSC-ex) may provide a promising strategy for treating liver injury; however, the role and underlying mechanisms of MSC-ex in steatosis are not fully understood.
Methods: Oleic-palmitic acid-treated hepatic cells and high-fat diet (HFD)-induced NAFLD mice were established to observe the effect of MSC-ex. Using non-targeted lipidomics and transcriptome analyses, we analysed the gene pathways positively correlated with MSC-ex. Mass spectrometry and gene knockdown/overexpression analyses were performed to evaluate the effect of calcium/calmodulin-dependent protein kinase 1 (CAMKK1) transferred by MSC-ex on lipid homoeostasis regulation.
Results: Here, we demonstrate that MSC-ex promote fatty acid oxidation and reduce lipogenesis in oleic-palmitic acid-treated hepatic cells and HFD-induced NAFLD mice. Non-targeted lipidomics and transcriptome analyses suggested that the effect of MSC-ex on lipid accumulation positively correlated with the phosphorylation of AMP-activated protein kinase. Furthermore, mass spectrometry and gene knockdown/overexpression analyses revealed that MSC-ex-transferred CAMKK1 is responsible for ameliorating lipid accumulation in an AMP-activated protein kinase-dependent manner, which subsequently inhibits SREBP-1C-mediated fatty acid synthesis and enhances peroxisome proliferator-activated receptor alpha (PPARα)-mediated fatty acid oxidation.
Conclusions: MSC-ex may prevent HFD-induced NAFLD via CAMKK1-mediated lipid homoeostasis regulation.
Impact and implications: NAFLD includes many conditions, from simple steatosis to non-alcoholic steatohepatitis, which can lead to fibrosis, cirrhosis, and even hepatocellular carcinoma. So far, there is no approved drug for treating liver steatosis of NAFLD. Thus, better therapies are needed to regulate lipid metabolism and prevent the progression from liver steatosis to chronic liver disease. By using a combination of non-targeted lipidomic and transcriptome analyses, we revealed that human umbilical cord mesenchymal stem cell-derived exosomes (MSC-ex) effectively reduced lipid deposition and improved liver function from HFD-induced liver steatosis. Our study highlights the importance of exosomal CAMKK1 from MSC-ex in mediating lipid metabolism regulation via AMPK-mediated PPARα/CPT-1A and SREBP-1C/fatty acid synthase signalling in hepatocytes. These findings are significant in elucidating novel mechanisms related to MSC-ex-based therapies for preventing NAFLD.
Keywords: AMPK; CAMKK1; Exosomes; MSC; NAFLD; Steatosis.
© 2023 The Authors.
Conflict of interest statement
The authors declare no competing financial interest. Please refer to the accompanying ICMJE disclosure forms for further details.
Figures
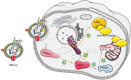
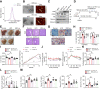
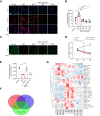
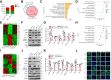
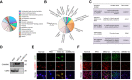
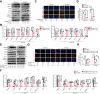
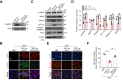
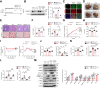
Similar articles
-
Tetrahydropalmatine ameliorates hepatic steatosis in nonalcoholic fatty liver disease by switching lipid metabolism via AMPK-SREBP-1c-Sirt1 signaling axis.Phytomedicine. 2023 Oct;119:155005. doi: 10.1016/j.phymed.2023.155005. Epub 2023 Aug 5. Phytomedicine. 2023. PMID: 37562090
-
Monascin and ankaflavin act as natural AMPK activators with PPARα agonist activity to down-regulate nonalcoholic steatohepatitis in high-fat diet-fed C57BL/6 mice.Food Chem Toxicol. 2014 Feb;64:94-103. doi: 10.1016/j.fct.2013.11.015. Epub 2013 Nov 22. Food Chem Toxicol. 2014. PMID: 24275089
-
Human umbilical cord-derived mesenchymal stem cell-exosomal miR-627-5p ameliorates non-alcoholic fatty liver disease by repressing FTO expression.Hum Cell. 2021 Nov;34(6):1697-1708. doi: 10.1007/s13577-021-00593-1. Epub 2021 Aug 19. Hum Cell. 2021. PMID: 34410623
-
Free radical biology for medicine: learning from nonalcoholic fatty liver disease.Free Radic Biol Med. 2013 Dec;65:952-968. doi: 10.1016/j.freeradbiomed.2013.08.174. Epub 2013 Aug 29. Free Radic Biol Med. 2013. PMID: 23994574 Review.
-
The ménage à trois of autophagy, lipid droplets and liver disease.Autophagy. 2022 Jan;18(1):50-72. doi: 10.1080/15548627.2021.1895658. Epub 2021 Apr 2. Autophagy. 2022. PMID: 33794741 Free PMC article. Review.
Cited by
-
Innovative mesenchymal stem cell treatments for fatty liver disease.World J Stem Cells. 2024 Sep 26;16(9):846-853. doi: 10.4252/wjsc.v16.i9.846. World J Stem Cells. 2024. PMID: 39351260 Free PMC article.
-
Adipose stem cells regulate lipid metabolism by upregulating mitochondrial fatty acid β-oxidation in macrophages to improve the retention rate of transplanted fat.Stem Cell Res Ther. 2024 Sep 27;15(1):328. doi: 10.1186/s13287-024-03953-4. Stem Cell Res Ther. 2024. PMID: 39334483 Free PMC article.
-
Mesenchymal stromal cells alleviate APAP-induced liver injury via extracellular vesicle-mediated regulation of the miR-186-5p/CXCL1 axis.Stem Cell Res Ther. 2024 Nov 3;15(1):392. doi: 10.1186/s13287-024-03995-8. Stem Cell Res Ther. 2024. PMID: 39490995 Free PMC article.
-
Mesenchymal Stem Cell-Derived Extracellular Vesicles Carrying Circ-Tulp4 Attenuate Diabetes Mellitus with Nonalcoholic Fatty Liver Disease by Inhibiting Cell Pyroptosis through the HNRNPC/ABHD6 Axis.Tissue Eng Regen Med. 2025 Jan;22(1):23-41. doi: 10.1007/s13770-024-00675-9. Epub 2024 Nov 15. Tissue Eng Regen Med. 2025. PMID: 39546192
-
Mechanism of mesenchymal stem cells in liver regeneration: Insights and future directions.World J Stem Cells. 2024 Sep 26;16(9):842-845. doi: 10.4252/wjsc.v16.i9.842. World J Stem Cells. 2024. PMID: 39351263 Free PMC article.
References
-
- Adams L.A., Waters O.R., Knuiman M.W., Elliott R.R., Olynyk J.K. NAFLD as a risk factor for the development of diabetes and the metabolic syndrome: an eleven-year follow-up study. Am J Gastroenterol. 2009;104:861–867. - PubMed
-
- Younossi Z., Anstee Q.M., Marietti M., Hardy T., Henry L., Eslam M., et al. Global burden of NAFLD and NASH: trends, predictions, risk factors and prevention. Nat Rev Gastroenterol Hepatol. 2018;15:11–20. - PubMed
-
- Radaelli M.G., Martucci F., Perra S., Accornero S., Castoldi G., Lattuada G., et al. NAFLD/NASH in patients with type 2 diabetes and related treatment options. J Endocrinol Invest. 2018;41:509–521. - PubMed
-
- Lee C.W., Hsiao W.T., Lee O.K. Mesenchymal stromal cell-based therapies reduce obesity and metabolic syndromes induced by a high-fat diet. Transl Res. 2017;182:61–74 e68. - PubMed
LinkOut - more resources
Full Text Sources