Thermoresponsive Polypeptide Fused L-Asparaginase with Mitigated Immunogenicity and Enhanced Efficacy in Treating Hematologic Malignancies
- PMID: 37271878
- PMCID: PMC10427413
- DOI: 10.1002/advs.202300469
Thermoresponsive Polypeptide Fused L-Asparaginase with Mitigated Immunogenicity and Enhanced Efficacy in Treating Hematologic Malignancies
Abstract
L-Asparaginase (ASP) is well-known for its excellent efficacy in treating hematological malignancies. Unfortunately, the intrinsic shortcomings of ASP, namely high immunogenicity, severe toxicity, short half-life, and poor stability, restrict its clinical usage. Poly(ethylene glycol) conjugation (PEGylation) of ASP is an effective strategy to address these issues, but it is not ideal in clinical applications due to complex chemical synthesis procedures, reduced ASP activity after conjugation, and pre-existing anti-PEG antibodies in humans. Herein, the authors genetically engineered an elastin-like polypeptide (ELP)-fused ASP (ASP-ELP), a core-shell structured tetramer predicted by AlphaFold2, to overcome the limitations of ASP and PEG-ASP. Notably, the unique thermosensitivity of ASP-ELP enables the in situ formation of a sustained-release depot post-injection with zero-order release kinetics over a long time. The in vitro and in vivo studies reveal that ASP-ELP possesses increased activity retention, improved stability, extended half-life, mitigated immunogenicity, reduced toxicity, and enhanced efficacy compared to ASP and PEG-ASP. Indeed, ASP-ELP treatment in leukemia or lymphoma mouse models of cell line-derived xenograft (CDX) shows potent anti-cancer effects with significantly prolonged survival. The findings also indicate that artificial intelligence (AI)-assisted genetic engineering is instructive in designing protein-polypeptide conjugates and may pave the way to develop next-generation biologics to enhance cancer treatment.
Keywords: L-asparaginase; artificial intelligence; elastin-like polypeptide; hematological malignancies; immunogenicity.
© 2023 The Authors. Advanced Science published by Wiley-VCH GmbH.
Conflict of interest statement
The authors declare no conflict of interest.
Figures
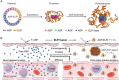
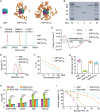
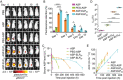
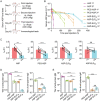
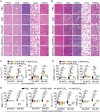
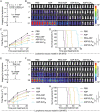
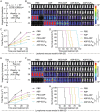
Similar articles
-
An ultra-long-acting L-asparaginase synergizes with an immune checkpoint inhibitor in starvation-immunotherapy of metastatic solid tumors.Biomaterials. 2025 Jan;312:122740. doi: 10.1016/j.biomaterials.2024.122740. Epub 2024 Jul 31. Biomaterials. 2025. PMID: 39096839
-
High efficacy of monomethoxypolyethylene glycol-conjugated L-asparaginase (PEG2-ASP) in two patients with hematological malignancies.Leuk Res. 1991;15(6):525-30. doi: 10.1016/0145-2126(91)90064-z. Leuk Res. 1991. PMID: 1861535
-
L-Asparaginase delivery systems targeted to minimize its side-effects.Adv Colloid Interface Sci. 2023 Jun;316:102915. doi: 10.1016/j.cis.2023.102915. Epub 2023 May 3. Adv Colloid Interface Sci. 2023. PMID: 37159987 Review.
-
Pegasparaginase: where do we stand?Expert Opin Biol Ther. 2009 Jan;9(1):111-9. doi: 10.1517/14712590802586058. Expert Opin Biol Ther. 2009. PMID: 19063697 Review.
-
L-asparaginase activity and anti-L-asparaginase antibody as biomarkers in estimating PEG-asp-related anaphylaxis risk in childhood acute lymphoblastic leukemia patients.Allergol Immunopathol (Madr). 2023 May 1;51(3):28-35. doi: 10.15586/aei.v51i3.771. eCollection 2023. Allergol Immunopathol (Madr). 2023. PMID: 37169557
Cited by
-
Substrate Affinity Is Not Crucial for Therapeutic L-Asparaginases: Antileukemic Activity of Novel Bacterial Enzymes.Molecules. 2024 May 11;29(10):2272. doi: 10.3390/molecules29102272. Molecules. 2024. PMID: 38792133 Free PMC article.
-
Protein-based nanoparticles for therapeutic nucleic acid delivery.Biomaterials. 2024 Mar;305:122464. doi: 10.1016/j.biomaterials.2023.122464. Epub 2024 Jan 2. Biomaterials. 2024. PMID: 38181574 Review.
-
Engineering and Expression Strategies for Optimization of L-Asparaginase Development and Production.Int J Mol Sci. 2023 Oct 16;24(20):15220. doi: 10.3390/ijms242015220. Int J Mol Sci. 2023. PMID: 37894901 Free PMC article. Review.
References
-
- a) Dores G. M., Curtis R. E., Dalal N. H., Linet M. S., Morton L. M., J. Clin. Oncol. 2020, 38, 4149; - PMC - PubMed
- b) Ferreyro B. L., Scales D. C., Wunsch H., Cheung M. C., Gupta V., Saskin R., Thyagu S., Munshi L., Intensive Care Med. 2021, 47, 1104; - PubMed
- c) Gray T. F., Temel J. S., El‐Jawahri A., Blood Rev. 2021, 45, 100692. - PubMed
-
- a) Chihara D., Huang E. P., Finnigan S. R., Cordes L. M., Skorupan N., Fukuda Y. K., Rubinstein L. V., Takebe N., Blood 2020, 136, 18;
- b) Craddock C., Friedberg J. W., J. Clin. Oncol. 2021, 39, 343; - PubMed
- c) Hou J. Z., Ye J. C., Pu J. J., Liu H., Ding W., Zheng H., Liu D., J. Hematol. Oncol. 2021, 14, 66; - PMC - PubMed
- d) Chihara D., Huang E. P., Finnigan S. R., Cordes L. M., Skorupan N., Fukuda Y., Rubinstein L. V., Ivy S. P., Doroshow J. H., Nastoupil L. J., Flowers C. R., Takebe N., J. Clin. Oncol. 2022, 40, 1949; - PMC - PubMed
- e) Finck A. V., Blanchard T., Roselle C. P., Golinelli G., June C. H., Nat. Med. 2022, 28, 678. - PMC - PubMed
-
- a) Larson R. C., Maus M. V., Nat. Rev. Cancer 2021, 21, 145; - PMC - PubMed
- b) Xue L., Yi Y., Xu Q., Wang L., Yang X., Zhang Y., Hua X., Chai X., Yang J., Chen Y., Tao G., Hu B., Wang X., Cell Discovery 2021, 7, 84; - PMC - PubMed
- c) Li F., Zhang H., Wang W., Yang P., Huang Y., Zhang J., Yan Y., Wang Y., Ding X., Liang J., Qi X., Li M., Han P., Zhang X., Wang X., Cao J., Fu Y. X., Yang X., Nat. Commun. 2022, 13, 4334; - PMC - PubMed
- d) Lu J., Jiang G., Mol. Cancer 2022, 21, 194. - PMC - PubMed
MeSH terms
Substances
Grants and funding
LinkOut - more resources
Full Text Sources