Challenges in studying the liquid-to-solid phase transitions of proteins using computer simulations
- PMID: 37267850
- PMCID: PMC10527940
- DOI: 10.1016/j.cbpa.2023.102333
Challenges in studying the liquid-to-solid phase transitions of proteins using computer simulations
Abstract
"Membraneless organelles," also referred to as biomolecular condensates, perform a variety of cellular functions and their dysregulation is implicated in cancer and neurodegeneration. In the last two decades, liquid-liquid phase separation (LLPS) of intrinsically disordered and multidomain proteins has emerged as a plausible mechanism underlying the formation of various biomolecular condensates. Further, the occurrence of liquid-to-solid transitions within liquid-like condensates may give rise to amyloid structures, implying a biophysical link between phase separation and protein aggregation. Despite significant advances, uncovering the microscopic details of liquid-to-solid phase transitions using experiments remains a considerable challenge and presents an exciting opportunity for the development of computational models which provide valuable, complementary insights into the underlying phenomenon. In this review, we first highlight recent biophysical studies which provide new insights into the molecular mechanisms underlying liquid-to-solid (fibril) phase transitions of folded, disordered and multi-domain proteins. Next, we summarize the range of computational models used to study protein aggregation and phase separation. Finally, we discuss recent computational approaches which attempt to capture the underlying physics of liquid-to-solid transitions along with their merits and shortcomings.
Keywords: Amyloid fibrils; Liquid-liquid phase separation (LLPS); Liquid-to-solid transition (LST); Molecular dynamics (MD) simulation.
Copyright © 2023 Elsevier Ltd. All rights reserved.
Conflict of interest statement
Declaration of competing interest The authors declare that they have no known competing financial interests or personal relationships that could have appeared to influence the work reported in this paper.
Figures
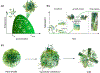
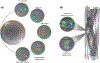
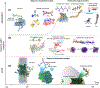
Similar articles
-
Unravelling the microscopic characteristics of intrinsically disordered proteins upon liquid-liquid phase separation.Essays Biochem. 2022 Dec 16;66(7):891-900. doi: 10.1042/EBC20220148. Essays Biochem. 2022. PMID: 36524527 Review.
-
Principles Governing the Phase Separation of Multidomain Proteins.Biochemistry. 2022 Nov 15;61(22):2443-2455. doi: 10.1021/acs.biochem.2c00210. Epub 2022 Jul 8. Biochemistry. 2022. PMID: 35802394 Free PMC article. Review.
-
Liquid-liquid phase separation of alpha-synuclein increases the structural variability of fibrils formed during amyloid aggregation.FEBS J. 2024 Oct;291(20):4522-4538. doi: 10.1111/febs.17244. Epub 2024 Aug 8. FEBS J. 2024. PMID: 39116032
-
Protein Condensates and Protein Aggregates: In Vitro, in the Cell, and In Silico.Front Biosci (Landmark Ed). 2023 Aug 28;28(8):183. doi: 10.31083/j.fbl2808183. Front Biosci (Landmark Ed). 2023. PMID: 37664947 Review.
-
Biological soft matter: intrinsically disordered proteins in liquid-liquid phase separation and biomolecular condensates.Essays Biochem. 2022 Dec 16;66(7):831-847. doi: 10.1042/EBC20220052. Essays Biochem. 2022. PMID: 36350034 Review.
Cited by
-
Interplay between charge distribution and DNA in shaping HP1 paralog phase separation and localization.Elife. 2024 Apr 9;12:RP90820. doi: 10.7554/eLife.90820. Elife. 2024. PMID: 38592759 Free PMC article.
-
A complex network of interdomain interactions underlies the conformational ensemble of monomeric TDP-43 and modulates its phase behavior.Protein Sci. 2024 Feb;33(2):e4891. doi: 10.1002/pro.4891. Protein Sci. 2024. PMID: 38160320 Free PMC article.
-
Expanding the molecular grammar of polar residues and arginine in FUS prion-like domain phase separation and aggregation.bioRxiv [Preprint]. 2024 Feb 15:2024.02.15.580391. doi: 10.1101/2024.02.15.580391. bioRxiv. 2024. PMID: 38405719 Free PMC article. Preprint.
References
-
- Ross CA and Poirier MA. What is the role of protein aggregation in neurodegeneration? Nat. Rev. Mol. Cell Biol, 6:891–898, 2005. - PubMed
-
- Alberti S and Hyman AA. Biomolecular condensates at the nexus of cellular stress, protein aggregation disease and ageing. Nat. Rev. Mol. Cell Biol, 22:196–213, 2021. - PubMed
-
- Shin Y and Brangwynne CP. Liquid phase condensation in cell physiology and disease. Science, 357:eaaf4382, 2017. - PubMed
Publication types
MeSH terms
Substances
Grants and funding
LinkOut - more resources
Full Text Sources