Construction of VSVΔ51M oncolytic virus expressing human interleukin-12
- PMID: 37255540
- PMCID: PMC10225647
- DOI: 10.3389/fmolb.2023.1190669
Construction of VSVΔ51M oncolytic virus expressing human interleukin-12
Abstract
The use of oncolytic viruses (OVs) in combination with cytokines, such as IL-12, is a promising approach for cancer treatment that addresses the limitations of current standard treatments and traditional cancer immunotherapies. IL-12, a proinflammatory cytokine, triggers intracellular signaling pathways that lead to increased apoptosis of tumor cells and enhanced antitumor activity of immune cells via IFN-γ induction, making this cytokine a promising candidate for cancer therapy. Targeted expression of IL-12 within tumors has been shown to play a crucial role in tumor eradication. The recent development of oncolytic viruses enables targeted delivery and expression of IL-12 at the tumor site, thereby addressing the systemic toxicities associated with traditional cancer therapy. In this study, we constructed an oncolytic virus, VSVΔ51M, based on the commercially available VSV wild-type backbone and further modified it to express human IL-12. Our preclinical data confirmed the safety and limited toxicity of the modified virus, VSV-Δ51M-hIL-12, supporting its potential use for clinical development.
Keywords: B16F10; MCF-7; VSVΔ51M; cancer immunotherapy; interleukin-12; oncolytic virus.
Copyright © 2023 Abdulal, Malki, Ghazal, Alsaieedi, Almahboub, Khan, Alsulaiman, Ghaith, Abujamel, Ganash, Mahmoud, Alkayyal and Hashem.
Conflict of interest statement
The authors declare that the research was conducted in the absence of any commercial or financial relationships that could be construed as a potential conflict of interest.
Figures
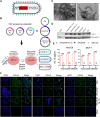
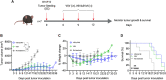
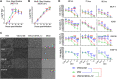
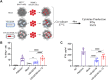
Similar articles
-
Intratumoral expression of interleukin 23 variants using oncolytic vaccinia virus elicit potent antitumor effects on multiple tumor models via tumor microenvironment modulation.Theranostics. 2021 May 3;11(14):6668-6681. doi: 10.7150/thno.56494. eCollection 2021. Theranostics. 2021. PMID: 34093846 Free PMC article.
-
Natural killer T cell immunotherapy combined with IL-15-expressing oncolytic virotherapy and PD-1 blockade mediates pancreatic tumor regression.J Immunother Cancer. 2022 Mar;10(3):e003923. doi: 10.1136/jitc-2021-003923. J Immunother Cancer. 2022. PMID: 35246474 Free PMC article.
-
Oncolytic Virus Encoding a Master Pro-Inflammatory Cytokine Interleukin 12 in Cancer Immunotherapy.Cells. 2020 Feb 10;9(2):400. doi: 10.3390/cells9020400. Cells. 2020. PMID: 32050597 Free PMC article. Review.
-
The efficacy versus toxicity profile of combination virotherapy and TLR immunotherapy highlights the danger of administering TLR agonists to oncolytic virus-treated mice.Mol Ther. 2013 Feb;21(2):348-57. doi: 10.1038/mt.2012.204. Epub 2012 Sep 25. Mol Ther. 2013. PMID: 23011032 Free PMC article.
-
Phase I study of VSV-GP (BI 1831169) as monotherapy or combined with ezabenlimab in advanced and refractory solid tumors.Future Oncol. 2022 Aug;18(24):2627-2638. doi: 10.2217/fon-2022-0439. Epub 2022 Jun 14. Future Oncol. 2022. PMID: 35699077 Review.
Cited by
-
A Method for the Production of Recombinant VSVs with Confirmation of Biological Activity.Acta Naturae. 2024 Jan-Mar;16(1):59-66. doi: 10.32607/actanaturae.27314. Acta Naturae. 2024. PMID: 38698956 Free PMC article.
References
-
- Abbott M., Ustoyev Y. (2019). “Cancer and the immune system: The history and background of immunotherapy,” in Seminars in oncology nursing (Netherlands: Elsevier; ). - PubMed
-
- Alkayyal A. A., Tai L. H., Kennedy M. A., de Souza C. T., Zhang J., Lefebvre C., et al. (2017). NK-cell recruitment is necessary for eradication of peritoneal carcinomatosis with an IL12-expressing maraba virus cellular vaccine. Cancer Immunol. Res. 5 (3), 211–221. 10.1158/2326-6066.CIR-16-0162 - DOI - PubMed
Grants and funding
LinkOut - more resources
Full Text Sources