Sequential Treatment with Temozolomide Plus Naturally Derived AT101 as an Alternative Therapeutic Strategy: Insights into Chemoresistance Mechanisms of Surviving Glioblastoma Cells
- PMID: 37240419
- PMCID: PMC10218802
- DOI: 10.3390/ijms24109075
Sequential Treatment with Temozolomide Plus Naturally Derived AT101 as an Alternative Therapeutic Strategy: Insights into Chemoresistance Mechanisms of Surviving Glioblastoma Cells
Abstract
Glioblastoma (GBM) is a poorly treatable disease due to the fast development of tumor recurrences and high resistance to chemo- and radiotherapy. To overcome the highly adaptive behavior of GBMs, especially multimodal therapeutic approaches also including natural adjuvants have been investigated. However, despite increased efficiency, some GBM cells are still able to survive these advanced treatment regimens. Given this, the present study evaluates representative chemoresistance mechanisms of surviving human GBM primary cells in a complex in vitro co-culture model upon sequential application of temozolomide (TMZ) combined with AT101, the R(-) enantiomer of the naturally occurring cottonseed-derived gossypol. Treatment with TMZ+AT101/AT101, although highly efficient, yielded a predominance of phosphatidylserine-positive GBM cells over time. Analysis of the intracellular effects revealed phosphorylation of AKT, mTOR, and GSK3ß, resulting in the induction of various pro-tumorigenic genes in surviving GBM cells. A Torin2-mediated mTOR inhibition combined with TMZ+AT101/AT101 partly counteracted the observed TMZ+AT101/AT101-associated effects. Interestingly, treatment with TMZ+AT101/AT101 concomitantly changed the amount and composition of extracellular vesicles released from surviving GBM cells. Taken together, our analyses revealed that even when chemotherapeutic agents with different effector mechanisms are combined, a variety of chemoresistance mechanisms of surviving GBM cells must be taken into account.
Keywords: AT101; R-(-)-gossypol; chemoresistance; combined therapy; epithelial–mesenchymal transition; glioblastoma; mTOR; stemness; temozolomide.
Conflict of interest statement
The authors declare no conflict of interest.
Figures
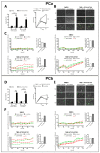
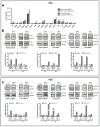
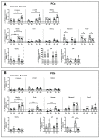
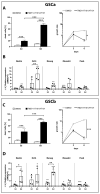
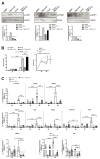
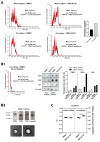
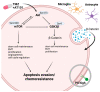
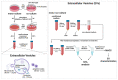
Similar articles
-
Effects of the Anti-Tumorigenic Agent AT101 on Human Glioblastoma Cells in the Microenvironmental Glioma Stem Cell Niche.Int J Mol Sci. 2021 Mar 30;22(7):3606. doi: 10.3390/ijms22073606. Int J Mol Sci. 2021. PMID: 33808494 Free PMC article.
-
Effects of sequentially applied single and combined temozolomide, hydroxychloroquine and AT101 treatment in a long-term stimulation glioblastoma in vitro model.J Cancer Res Clin Oncol. 2018 Aug;144(8):1475-1485. doi: 10.1007/s00432-018-2680-y. Epub 2018 Jun 1. J Cancer Res Clin Oncol. 2018. PMID: 29858681
-
Combined treatment of AT101 and demethoxycurcumin yields an enhanced anti-proliferative effect in human primary glioblastoma cells.J Cancer Res Clin Oncol. 2020 Jan;146(1):117-126. doi: 10.1007/s00432-019-03107-7. Epub 2019 Dec 16. J Cancer Res Clin Oncol. 2020. PMID: 31844979
-
Involvement of Intracellular Cholesterol in Temozolomide-Induced Glioblastoma Cell Death.Neurol Med Chir (Tokyo). 2018 Jul 15;58(7):296-302. doi: 10.2176/nmc.ra.2018-0040. Epub 2018 Jun 13. Neurol Med Chir (Tokyo). 2018. PMID: 29899179 Free PMC article. Review.
-
Dysregulated lipid metabolism in TMZ-resistant glioblastoma: pathways, proteins, metabolites and therapeutic opportunities.Lipids Health Dis. 2023 Aug 3;22(1):114. doi: 10.1186/s12944-023-01881-5. Lipids Health Dis. 2023. PMID: 37537607 Free PMC article. Review.
Cited by
-
Three-Dimensional Hepatocyte Spheroids: Model for Assessing Chemotherapy in Hepatocellular Carcinoma.Biomedicines. 2024 May 28;12(6):1200. doi: 10.3390/biomedicines12061200. Biomedicines. 2024. PMID: 38927406 Free PMC article.
References
-
- Verhaak R.G.W., Hoadley K.A., Purdom E., Wang V., Wilkerson M.D., Miller C.R., Ding L., Golub T., Jill P., Alexe G., et al. Integrated Genomic Analysis Identifies Clinically Relevant Subtypes of Glioblastoma Characterized by Abnormalities in PDGFRA, IDH1, EGFR, and NF1. Cancer Cell. 2010;17:98–110. doi: 10.1016/j.ccr.2009.12.020. - DOI - PMC - PubMed
MeSH terms
Substances
Grants and funding
LinkOut - more resources
Full Text Sources
Medical
Miscellaneous