Spike-Independent Infection of Human Coronavirus 229E in Bat Cells
- PMID: 37199653
- PMCID: PMC10269751
- DOI: 10.1128/spectrum.03483-22
Spike-Independent Infection of Human Coronavirus 229E in Bat Cells
Abstract
Bats are the reservoir for numerous human pathogens, including coronaviruses. Despite many coronaviruses having descended from bat ancestors, little is known about virus-host interactions and broader evolutionary history involving bats. Studies have largely focused on the zoonotic potential of coronaviruses with few infection experiments conducted in bat cells. To determine genetic changes derived from replication in bat cells and possibly identify potential novel evolutionary pathways for zoonotic virus emergence, we serially passaged six human 229E isolates in a newly established Rhinolophus lepidus (horseshoe bat) kidney cell line. Here, we observed extensive deletions within the spike and open reading frame 4 (ORF4) genes of five 229E viruses after passaging in bat cells. As a result, spike protein expression and infectivity of human cells was lost in 5 of 6 viruses, but the capability to infect bat cells was maintained. Only viruses that expressed the spike protein could be neutralized by 229E spike-specific antibodies in human cells, whereas there was no neutralizing effect on viruses that did not express the spike protein inoculated on bat cells. However, one isolate acquired an early stop codon, abrogating spike expression but maintaining infection in bat cells. After passaging this isolate in human cells, spike expression was restored due to acquisition of nucleotide insertions among virus subpopulations. Spike-independent infection of human coronavirus 229E may provide an alternative mechanism for viral maintenance in bats that does not rely on the compatibility of viral surface proteins and known cellular entry receptors. IMPORTANCE Many viruses, including coronaviruses, originated from bats. Yet, we know little about how these viruses switch between hosts and enter human populations. Coronaviruses have succeeded in establishing in humans at least five times, including endemic coronaviruses and the recent severe acute respiratory syndrome coronavirus 2 (SARS-CoV-2). In an approach to identify requirements for host switches, we established a bat cell line and adapted human coronavirus 229E viruses by serial passage. The resulting viruses lost their spike protein but maintained the ability to infect bat cells, but not human cells. Maintenance of 229E viruses in bat cells appears to be independent of a canonical spike receptor match, which in turn might facilitate cross-species transmission in bats.
Keywords: 229E; coronavirus; evolution; pandemic; receptor; receptor usage; spike; zoonotic.
Conflict of interest statement
The authors declare no conflict of interest.
Figures
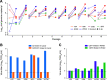
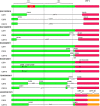
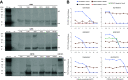
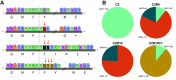
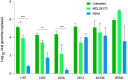
Similar articles
-
Evidence for an Ancestral Association of Human Coronavirus 229E with Bats.J Virol. 2015 Dec;89(23):11858-70. doi: 10.1128/JVI.01755-15. Epub 2015 Sep 16. J Virol. 2015. PMID: 26378164 Free PMC article.
-
Surveillance of Bat Coronaviruses in Kenya Identifies Relatives of Human Coronaviruses NL63 and 229E and Their Recombination History.J Virol. 2017 Feb 14;91(5):e01953-16. doi: 10.1128/JVI.01953-16. Print 2017 Mar 1. J Virol. 2017. PMID: 28077633 Free PMC article.
-
Evolutionary Arms Race between Virus and Host Drives Genetic Diversity in Bat Severe Acute Respiratory Syndrome-Related Coronavirus Spike Genes.J Virol. 2020 Sep 29;94(20):e00902-20. doi: 10.1128/JVI.00902-20. Print 2020 Sep 29. J Virol. 2020. PMID: 32699095 Free PMC article.
-
Properties of Coronavirus and SARS-CoV-2.Malays J Pathol. 2020 Apr;42(1):3-11. Malays J Pathol. 2020. PMID: 32342926 Review.
-
[Source of the COVID-19 pandemic: ecology and genetics of coronaviruses (Betacoronavirus: Coronaviridae) SARS-CoV, SARS-CoV-2 (subgenus Sarbecovirus), and MERS-CoV (subgenus Merbecovirus).].Vopr Virusol. 2020;65(2):62-70. doi: 10.36233/0507-4088-2020-65-2-62-70. Vopr Virusol. 2020. PMID: 32515561 Review. Russian.
Cited by
-
Positive-strand RNA viruses-a Keystone Symposia report.Ann N Y Acad Sci. 2023 Mar;1521(1):46-66. doi: 10.1111/nyas.14957. Epub 2023 Jan 25. Ann N Y Acad Sci. 2023. PMID: 36697369 Free PMC article.
-
A Look inside the Replication Dynamics of SARS-CoV-2 in Blyth's Horseshoe Bat (Rhinolophus lepidus) Kidney Cells.Microbiol Spectr. 2022 Jun 29;10(3):e0044922. doi: 10.1128/spectrum.00449-22. Epub 2022 May 31. Microbiol Spectr. 2022. PMID: 35638834 Free PMC article.
References
-
- Gorbalenya AE, Baker SC, Baric RS, de Groot RJ, Drosten C, Gulyaeva AA, Haagmans BL, Lauber C, Leontovich AM, Neuman BW, Penzar D, Perlman S, Poon LLM, Samborskiy DV, Sidorov IA, Sola I, Ziebuhr J. Coronaviridae Study Group of the International Committee on Taxonomy of Viruses. 2020. The species severe acute respiratory syndrome-related coronavirus: classifying 2019-nCoV and naming it SARS-CoV-2. Nat Microbiol 5:536–544. doi:10.1038/s41564-020-0695-z. - DOI - PMC - PubMed
-
- Peiris JSM, Lai ST, Poon LLM, Guan Y, Yam LYC, Lim W, Nicholls J, Yee WKS, Yan WW, Cheung MT, Cheng VCC, Chan KH, Tsang DNC, Yung RWH, Ng TK, Yuen KY. SARS study group. 2003. Coronavirus as a possible cause of severe acute respiratory syndrome. Lancet 361:1319–1325. doi:10.1016/S0140-6736(03)13077-2. - DOI - PMC - PubMed
-
- Ksiazek TG, Erdman D, Goldsmith CS, Zaki SR, Peret T, Emery S, Tong S, Urbani C, Comer JA, Lim W, Rollin PE, Dowell SF, Ling A-E, Humphrey CD, Shieh W-J, Guarner J, Paddock CD, Rota P, Fields B, DeRisi J, Yang J-Y, Cox N, Hughes JM, LeDuc JW, Bellini WJ, Anderson LJ. SARS Working Group. 2003. A novel coronavirus associated with severe acute respiratory syndrome. N Engl J Med 348:1953–1966. doi:10.1056/NEJMoa030781. - DOI - PubMed
Publication types
MeSH terms
Substances
LinkOut - more resources
Full Text Sources
Medical
Research Materials
Miscellaneous