The C10orf76-PI4KB axis orchestrates CERT-mediated ceramide trafficking to the distal Golgi
- PMID: 37195633
- PMCID: PMC10192306
- DOI: 10.1083/jcb.202111069
The C10orf76-PI4KB axis orchestrates CERT-mediated ceramide trafficking to the distal Golgi
Abstract
Phosphatidylinositol 4-monophosphate [PtdIns(4)P] is a precursor for various phosphoinositides but also a membrane-embedded component crucial for membrane contact sites (MCSs). Several lipid transfer proteins are recruited to MCSs by recognizing PtdIns(4)P; however, it remains poorly elucidated how the production of PtdIns(4)P for lipid transport at MCSs is regulated. Following human genome-wide screening, we discovered that the PtdIns(4)P-related genes PI4KB, ACBD3, and C10orf76 are involved in endoplasmic reticulum-to-Golgi trafficking of ceramide by the ceramide transport protein CERT. CERT preferentially utilizes PtdIns(4)P generated by PI4KB recruited to the Golgi by C10orf76 rather than by ACBD3. Super-resolution microscopy observation revealed that C10orf76 predominantly localizes at distal Golgi regions, where sphingomyelin (SM) synthesis primarily occurs, while the majority of ACBD3 localizes at more proximal regions. This study provides a proof-of-concept that distinct pools of PtdIns(4)P are generated in different subregions, even within the same organelle, to facilitate interorganelle metabolic channeling for the ceramide-to-SM conversion.
© 2023 Mizuike et al.
Conflict of interest statement
Disclosures: The authors declare no competing interests exist.
Figures
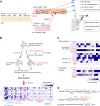
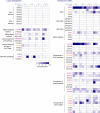
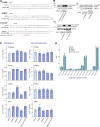
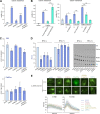
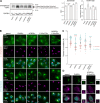
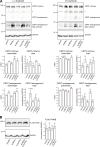
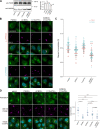
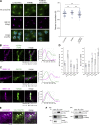
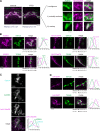
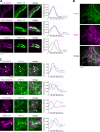
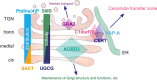
Similar articles
-
DGARM/C10orf76/ARMH3 for Ceramide Transfer Zone at the Endoplasmic Reticulum-Distal Golgi Contacts.Contact (Thousand Oaks). 2024 Mar 20;7:25152564241239443. doi: 10.1177/25152564241239443. eCollection 2024 Jan-Dec. Contact (Thousand Oaks). 2024. PMID: 38515862 Free PMC article. Review.
-
Structure, functions and regulation of CERT, a lipid-transfer protein for the delivery of ceramide at the ER-Golgi membrane contact sites.FEBS Lett. 2019 Sep;593(17):2366-2377. doi: 10.1002/1873-3468.13511. Epub 2019 Jul 8. FEBS Lett. 2019. PMID: 31254361 Review.
-
Involvement of a Cluster of Basic Amino Acids in Phosphorylation-Dependent Functional Repression of the Ceramide Transport Protein CERT.Int J Mol Sci. 2022 Aug 2;23(15):8576. doi: 10.3390/ijms23158576. Int J Mol Sci. 2022. PMID: 35955719 Free PMC article.
-
Interaction between the PH and START domains of ceramide transfer protein competes with phosphatidylinositol 4-phosphate binding by the PH domain.J Biol Chem. 2017 Aug 25;292(34):14217-14228. doi: 10.1074/jbc.M117.780007. Epub 2017 Jun 26. J Biol Chem. 2017. PMID: 28652409 Free PMC article.
-
CERT-mediated trafficking of ceramide.Biochim Biophys Acta. 2009 Jul;1791(7):684-91. doi: 10.1016/j.bbalip.2009.01.006. Epub 2009 Jan 22. Biochim Biophys Acta. 2009. PMID: 19416656 Review.
Cited by
-
The Implication of Sphingolipids in Viral Infections.Int J Mol Sci. 2023 Dec 9;24(24):17303. doi: 10.3390/ijms242417303. Int J Mol Sci. 2023. PMID: 38139132 Free PMC article. Review.
-
ARMH3 is an ARL5 effector that promotes PI4KB-catalyzed PI4P synthesis at the trans-Golgi network.Nat Commun. 2024 Nov 23;15(1):10168. doi: 10.1038/s41467-024-54410-y. Nat Commun. 2024. PMID: 39580461 Free PMC article.
-
Mechanisms of Nonvesicular Ceramide Transport.Contact (Thousand Oaks). 2023 Oct 17;6:25152564231208250. doi: 10.1177/25152564231208250. eCollection 2023 Jan-Dec. Contact (Thousand Oaks). 2023. PMID: 37859671 Free PMC article. Review.
-
A Membrane-Assisted Mechanism for the Release of Ceramide from the CERT START Domain.J Phys Chem B. 2024 Jul 4;128(26):6338-6351. doi: 10.1021/acs.jpcb.4c02398. Epub 2024 Jun 21. J Phys Chem B. 2024. PMID: 38903016 Free PMC article.
-
DGARM/C10orf76/ARMH3 for Ceramide Transfer Zone at the Endoplasmic Reticulum-Distal Golgi Contacts.Contact (Thousand Oaks). 2024 Mar 20;7:25152564241239443. doi: 10.1177/25152564241239443. eCollection 2024 Jan-Dec. Contact (Thousand Oaks). 2024. PMID: 38515862 Free PMC article. Review.
References
Publication types
MeSH terms
Substances
LinkOut - more resources
Full Text Sources
Molecular Biology Databases