The asymmetrical ESR1 signaling in muscle progenitor cells determines the progression of adolescent idiopathic scoliosis
- PMID: 37185898
- PMCID: PMC10130095
- DOI: 10.1038/s41421-023-00531-5
The asymmetrical ESR1 signaling in muscle progenitor cells determines the progression of adolescent idiopathic scoliosis
Abstract
Adolescent Idiopathic Scoliosis (AIS) is a common pediatric skeletal disease highly occurred in females. The pathogenesis of AIS has not been fully elucidated. Here, we reveal that ESR1 (Estrogen Receptor 1) expression declines in muscle stem/progenitor cells at the concave side of AIS patients. Furthermore, ESR1 is required for muscle stem/progenitor cell differentiation and disrupted ESR1 signaling leads to differentiation defects. The imbalance of ESR1 signaling in the para-spinal muscles induces scoliosis in mice, while reactivation of ESR1 signaling at the concave side by an FDA approved drug Raloxifene alleviates the curve progression. This work reveals that the asymmetric inactivation of ESR1 signaling is one of the causes of AIS. Reactivation of ESR1 signaling in para-spinal muscle by Raloxifene at the concave side could be a new strategy to treat AIS.
© 2023. The Author(s).
Conflict of interest statement
The authors declare no competing interests.
Figures
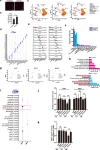
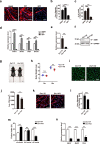
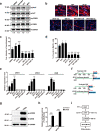
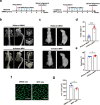
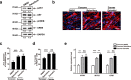
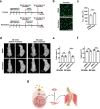
Similar articles
-
Paraspinal muscle ladybird homeobox 1 (LBX1) in adolescent idiopathic scoliosis: a cross-sectional study.Spine J. 2019 Dec;19(12):1911-1916. doi: 10.1016/j.spinee.2019.06.014. Epub 2019 Jun 14. Spine J. 2019. PMID: 31202838
-
A relook into the association of the estrogen receptor [alpha] gene (PvuII, XbaI) and adolescent idiopathic scoliosis: a study of 540 Chinese cases.Spine (Phila Pa 1976). 2006 Oct 1;31(21):2463-8. doi: 10.1097/01.brs.0000239179.81596.2b. Spine (Phila Pa 1976). 2006. PMID: 17023856
-
Methylation of Estrogen Receptor 1 Gene in the Paraspinal Muscles of Girls with Idiopathic Scoliosis and Its Association with Disease Severity.Genes (Basel). 2021 May 21;12(6):790. doi: 10.3390/genes12060790. Genes (Basel). 2021. PMID: 34064195 Free PMC article.
-
Is spinal neuromuscular function asymmetrical in adolescents with idiopathic scoliosis compared to those without scoliosis?: A narrative review of surface EMG studies.J Electromyogr Kinesiol. 2022 Apr;63:102640. doi: 10.1016/j.jelekin.2022.102640. Epub 2022 Feb 7. J Electromyogr Kinesiol. 2022. PMID: 35219074 Review.
-
Predictive value of single-nucleotide polymorphisms in curve progression of adolescent idiopathic scoliosis.Eur Spine J. 2022 Sep;31(9):2311-2325. doi: 10.1007/s00586-022-07213-y. Epub 2022 Apr 17. Eur Spine J. 2022. PMID: 35434775 Review.
Cited by
-
Spinal muscle characteristics during three different types of locomotion activities among college students with idiopathic scoliosis.BMC Musculoskelet Disord. 2024 Oct 29;25(1):862. doi: 10.1186/s12891-024-07954-5. BMC Musculoskelet Disord. 2024. PMID: 39472828 Free PMC article.
-
Diabetes mellitus in breast cancer survivors: metabolic effects of endocrine therapy.Nat Rev Endocrinol. 2024 Jan;20(1):16-26. doi: 10.1038/s41574-023-00899-0. Epub 2023 Oct 2. Nat Rev Endocrinol. 2024. PMID: 37783846 Free PMC article. Review.
-
Factors that influence in-brace derotation effects in patients with adolescent idiopathic scoliosis: a study based on EOS imaging system.J Orthop Surg Res. 2024 May 12;19(1):293. doi: 10.1186/s13018-024-04789-7. J Orthop Surg Res. 2024. PMID: 38735944 Free PMC article.
-
The reciprocity of skeletal muscle and bone: an evolving view from mechanical coupling, secretory crosstalk to stem cell exchange.Front Physiol. 2024 Mar 4;15:1349253. doi: 10.3389/fphys.2024.1349253. eCollection 2024. Front Physiol. 2024. PMID: 38505709 Free PMC article. Review.
-
Comparative analysis of paraspinal muscle imbalance between idiopathic scoliosis and congenital scoliosis from the transcriptome aspect.JOR Spine. 2024 Mar 4;7(1):e1318. doi: 10.1002/jsp2.1318. eCollection 2024 Mar. JOR Spine. 2024. PMID: 38440359 Free PMC article.
References
Grants and funding
LinkOut - more resources
Full Text Sources
Research Materials
Miscellaneous