Dynamic conformational switching underlies TFIIH function in transcription and DNA repair and impacts genetic diseases
- PMID: 37179334
- PMCID: PMC10183003
- DOI: 10.1038/s41467-023-38416-6
Dynamic conformational switching underlies TFIIH function in transcription and DNA repair and impacts genetic diseases
Abstract
Transcription factor IIH (TFIIH) is a protein assembly essential for transcription initiation and nucleotide excision repair (NER). Yet, understanding of the conformational switching underpinning these diverse TFIIH functions remains fragmentary. TFIIH mechanisms critically depend on two translocase subunits, XPB and XPD. To unravel their functions and regulation, we build cryo-EM based TFIIH models in transcription- and NER-competent states. Using simulations and graph-theoretical analysis methods, we reveal TFIIH's global motions, define TFIIH partitioning into dynamic communities and show how TFIIH reshapes itself and self-regulates depending on functional context. Our study uncovers an internal regulatory mechanism that switches XPB and XPD activities making them mutually exclusive between NER and transcription initiation. By sequentially coordinating the XPB and XPD DNA-unwinding activities, the switch ensures precise DNA incision in NER. Mapping TFIIH disease mutations onto network models reveals clustering into distinct mechanistic classes, affecting translocase functions, protein interactions and interface dynamics.
© 2023. The Author(s).
Conflict of interest statement
The authors declare no competing interests.
Figures
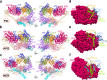
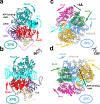
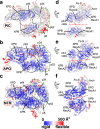
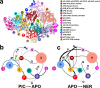
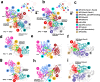
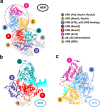
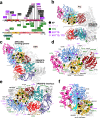
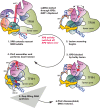
Similar articles
-
XPB and XPD helicases in TFIIH orchestrate DNA duplex opening and damage verification to coordinate repair with transcription and cell cycle via CAK kinase.DNA Repair (Amst). 2011 Jul 15;10(7):697-713. doi: 10.1016/j.dnarep.2011.04.028. Epub 2011 May 14. DNA Repair (Amst). 2011. PMID: 21571596 Free PMC article. Review.
-
Structural basis of TFIIH activation for nucleotide excision repair.Nat Commun. 2019 Jun 28;10(1):2885. doi: 10.1038/s41467-019-10745-5. Nat Commun. 2019. PMID: 31253769 Free PMC article.
-
The long unwinding road: XPB and XPD helicases in damaged DNA opening.Cell Cycle. 2010 Jan 1;9(1):90-6. doi: 10.4161/cc.9.1.10267. Epub 2010 Jan 5. Cell Cycle. 2010. PMID: 20016270 Review.
-
Envisioning how the prototypic molecular machine TFIIH functions in transcription initiation and DNA repair.DNA Repair (Amst). 2020 Dec;96:102972. doi: 10.1016/j.dnarep.2020.102972. Epub 2020 Sep 17. DNA Repair (Amst). 2020. PMID: 33007515 Free PMC article. Review.
-
TFIIH central activity in nucleotide excision repair to prevent disease.DNA Repair (Amst). 2023 Dec;132:103568. doi: 10.1016/j.dnarep.2023.103568. Epub 2023 Sep 7. DNA Repair (Amst). 2023. PMID: 37977600 Review.
Cited by
-
Uncoupling the TFIIH Core and Kinase Modules Leads To Misregulated RNA Polymerase II CTD Serine 5 Phosphorylation.bioRxiv [Preprint]. 2024 Jun 13:2023.09.11.557269. doi: 10.1101/2023.09.11.557269. bioRxiv. 2024. PMID: 37745343 Free PMC article. Preprint.
-
Differing structures and dynamics of two photolesions portray verification differences by the human XPD helicase.Nucleic Acids Res. 2023 Dec 11;51(22):12261-12274. doi: 10.1093/nar/gkad974. Nucleic Acids Res. 2023. PMID: 37933861 Free PMC article.
-
Glyoxal oxidase-mediated detoxification of reactive carbonyl species contributes to virulence, stress tolerance, and development in a pathogenic fungus.PLoS Pathog. 2024 Jul 30;20(7):e1012431. doi: 10.1371/journal.ppat.1012431. eCollection 2024 Jul. PLoS Pathog. 2024. PMID: 39078845 Free PMC article.
-
Molecular architecture and functional dynamics of the pre-incision complex in nucleotide excision repair.Nat Commun. 2024 Oct 1;15(1):8511. doi: 10.1038/s41467-024-52860-y. Nat Commun. 2024. PMID: 39353945 Free PMC article.