Structure of the endosomal Commander complex linked to Ritscher-Schinzel syndrome
- PMID: 37172566
- PMCID: PMC10187114
- DOI: 10.1016/j.cell.2023.04.003
Structure of the endosomal Commander complex linked to Ritscher-Schinzel syndrome
Abstract
The Commander complex is required for endosomal recycling of diverse transmembrane cargos and is mutated in Ritscher-Schinzel syndrome. It comprises two sub-assemblies: Retriever composed of VPS35L, VPS26C, and VPS29; and the CCC complex which contains twelve subunits: COMMD1-COMMD10 and the coiled-coil domain-containing (CCDC) proteins CCDC22 and CCDC93. Combining X-ray crystallography, electron cryomicroscopy, and in silico predictions, we have assembled a complete structural model of Commander. Retriever is distantly related to the endosomal Retromer complex but has unique features preventing the shared VPS29 subunit from interacting with Retromer-associated factors. The COMMD proteins form a distinctive hetero-decameric ring stabilized by extensive interactions with CCDC22 and CCDC93. These adopt a coiled-coil structure that connects the CCC and Retriever assemblies and recruits a 16th subunit, DENND10, to form the complete Commander complex. The structure allows mapping of disease-causing mutations and reveals the molecular features required for the function of this evolutionarily conserved trafficking machinery.
Keywords: AlphaFold; CCC complex; CCDC22; CCDC93; COMMD; Commander; DENND10; Endosome; Retriever; Retromer; Ritscher-Schinzel syndrome; VPS29.
Copyright © 2023 The Author(s). Published by Elsevier Inc. All rights reserved.
Conflict of interest statement
Declaration of interests The authors declare that they have no conflicts of interest.
Figures
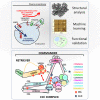
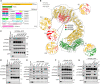
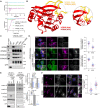
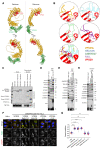
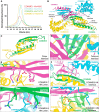
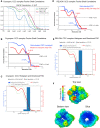
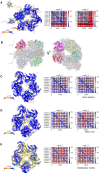
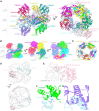
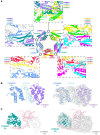
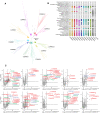
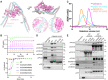
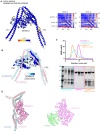

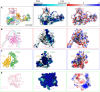
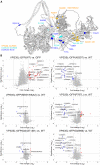
Comment in
-
Commanding the Commander: structure of a key protein machinery in endosomal trafficking.Signal Transduct Target Ther. 2023 Aug 4;8(1):295. doi: 10.1038/s41392-023-01568-4. Signal Transduct Target Ther. 2023. PMID: 37542035 Free PMC article. No abstract available.
Similar articles
-
Depressing time: Waiting, melancholia, and the psychoanalytic practice of care.In: Kirtsoglou E, Simpson B, editors. The Time of Anthropology: Studies of Contemporary Chronopolitics. Abingdon: Routledge; 2020. Chapter 5. In: Kirtsoglou E, Simpson B, editors. The Time of Anthropology: Studies of Contemporary Chronopolitics. Abingdon: Routledge; 2020. Chapter 5. PMID: 36137063 Free Books & Documents. Review.
-
Unlocking data: Decision-maker perspectives on cross-sectoral data sharing and linkage as part of a whole-systems approach to public health policy and practice.Public Health Res (Southampt). 2024 Nov 20:1-30. doi: 10.3310/KYTW2173. Online ahead of print. Public Health Res (Southampt). 2024. PMID: 39582242
-
Comparison of Two Modern Survival Prediction Tools, SORG-MLA and METSSS, in Patients With Symptomatic Long-bone Metastases Who Underwent Local Treatment With Surgery Followed by Radiotherapy and With Radiotherapy Alone.Clin Orthop Relat Res. 2024 Dec 1;482(12):2193-2208. doi: 10.1097/CORR.0000000000003185. Epub 2024 Jul 23. Clin Orthop Relat Res. 2024. PMID: 39051924
-
Qualitative evidence synthesis informing our understanding of people's perceptions and experiences of targeted digital communication.Cochrane Database Syst Rev. 2019 Oct 23;10(10):ED000141. doi: 10.1002/14651858.ED000141. Cochrane Database Syst Rev. 2019. PMID: 31643081 Free PMC article.
-
Pharmacological treatments in panic disorder in adults: a network meta-analysis.Cochrane Database Syst Rev. 2023 Nov 28;11(11):CD012729. doi: 10.1002/14651858.CD012729.pub3. Cochrane Database Syst Rev. 2023. PMID: 38014714 Free PMC article. Review.
Cited by
-
Commanding the Commander: structure of a key protein machinery in endosomal trafficking.Signal Transduct Target Ther. 2023 Aug 4;8(1):295. doi: 10.1038/s41392-023-01568-4. Signal Transduct Target Ther. 2023. PMID: 37542035 Free PMC article. No abstract available.
-
Structural Organization of the Retriever-CCC Endosomal Recycling Complex.Res Sq [Preprint]. 2023 Jun 16:rs.3.rs-3026818. doi: 10.21203/rs.3.rs-3026818/v1. Res Sq. 2023. Update in: Nat Struct Mol Biol. 2024 Jun;31(6):910-924. doi: 10.1038/s41594-023-01184-4 PMID: 37397996 Free PMC article. Updated. Preprint.
-
COMMD10 inhibited DNA damage to promote the progression of gastric cancer.J Cancer Res Clin Oncol. 2024 Jun 13;150(6):305. doi: 10.1007/s00432-024-05817-z. J Cancer Res Clin Oncol. 2024. PMID: 38871970 Free PMC article.
-
Structural Organization of the Retriever-CCC Endosomal Recycling Complex.bioRxiv [Preprint]. 2023 Jun 7:2023.06.06.543888. doi: 10.1101/2023.06.06.543888. bioRxiv. 2023. Update in: Nat Struct Mol Biol. 2024 Jun;31(6):910-924. doi: 10.1038/s41594-023-01184-4 PMID: 37333304 Free PMC article. Updated. Preprint.
-
Structure and interactions of the endogenous human Commander complex.Nat Struct Mol Biol. 2024 Jun;31(6):925-938. doi: 10.1038/s41594-024-01246-1. Epub 2024 Mar 8. Nat Struct Mol Biol. 2024. PMID: 38459129 Free PMC article.
References
Publication types
MeSH terms
Substances
Supplementary concepts
Grants and funding
LinkOut - more resources
Full Text Sources
Medical
Research Materials