Trichloroethylene Metabolite S-(1,2-Dichlorovinyl)-l-cysteine Stimulates Changes in Energy Metabolites and Amino Acids in the BeWo Human Placental Trophoblast Model during Syncytialization
- PMID: 37162359
- PMCID: PMC10499396
- DOI: 10.1021/acs.chemrestox.3c00007
Trichloroethylene Metabolite S-(1,2-Dichlorovinyl)-l-cysteine Stimulates Changes in Energy Metabolites and Amino Acids in the BeWo Human Placental Trophoblast Model during Syncytialization
Abstract
Syncytialization, the fusion of cytotrophoblasts into an epithelial barrier that constitutes the maternal-fetal interface, is a crucial event of placentation. This process is characterized by distinct changes to amino acid and energy metabolism. A metabolite of the industrial solvent trichloroethylene (TCE), S-(1,2-dichlorovinyl)-l-cysteine (DCVC), modifies energy metabolism and amino acid abundance in HTR-8/SVneo extravillous trophoblasts. In the current study, we investigated DCVC-induced changes to energy metabolism and amino acids during forskolin-stimulated syncytialization in BeWo cells, a human villous trophoblastic cell line that models syncytialization in vitro. BeWo cells were exposed to forskolin at 100 μM for 48 h to stimulate syncytialization. During syncytialization, BeWo cells were also treated with DCVC at 0 (control), 10, or 20 μM. Following treatment, the targeted metabolomics platform, "Tricarboxylic Acid Plus", was used to identify changes in energy metabolism and amino acids. DCVC treatment during syncytialization decreased oleic acid, aspartate, proline, uridine diphosphate (UDP), UDP-d-glucose, uridine monophosphate, and cytidine monophosphate relative to forskolin-only treatment controls, but did not increase any measured metabolite. Notable changes stimulated by syncytialization in the absence of DCVC included increased adenosine monophosphate and guanosine monophosphate, as well as decreased aspartate and glutamate. Pathway analysis revealed multiple pathways in amino acid and sugar metabolisms that were altered with forskolin-stimulated syncytialization alone and DCVC treatment during syncytialization. Analysis of ratios of metabolites within the pathways revealed that DCVC exposure during syncytialization changed metabolite ratios in the same or different direction compared to syncytialization alone. Building off our oleic acid findings, we found that extracellular matrix metalloproteinase-2, which is downstream in oleic acid signaling, underwent the same changes as oleic acid. Together, the metabolic changes stimulated by DCVC treatment during syncytialization suggest changes in energy metabolism and amino acid abundance as potential mechanisms by which DCVC could impact syncytialization and pregnancy.
Conflict of interest statement
The authors declare no competing financial interest.
Figures
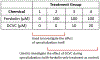
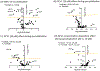
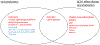
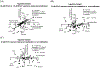
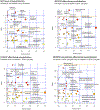
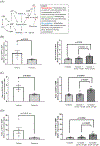
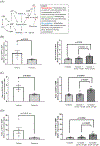
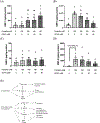
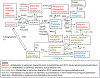
Similar articles
-
N-Acetyl-L-cysteine and aminooxyacetic acid differentially modulate toxicity of the trichloroethylene metabolite S-(1,2-dichlorovinyl)-L-cysteine in human placental villous trophoblast BeWo cells.Toxicology. 2023 Aug 15;495:153611. doi: 10.1016/j.tox.2023.153611. Epub 2023 Aug 5. Toxicology. 2023. PMID: 37544576 Free PMC article.
-
Apoptotic responses stimulated by the trichloroethylene metabolite S-(1,2-dichlorovinyl)-L-cysteine depend on cell differentiation state in BeWo human trophoblast cells.Toxicol In Vitro. 2023 Feb;86:105514. doi: 10.1016/j.tiv.2022.105514. Epub 2022 Nov 4. Toxicol In Vitro. 2023. PMID: 36336211 Free PMC article.
-
Exposure to Trichloroethylene Metabolite S-(1,2-Dichlorovinyl)-L-cysteine Causes Compensatory Changes to Macronutrient Utilization and Energy Metabolism in Placental HTR-8/SVneo Cells.Chem Res Toxicol. 2020 Jun 15;33(6):1339-1355. doi: 10.1021/acs.chemrestox.9b00356. Epub 2020 Jan 30. Chem Res Toxicol. 2020. PMID: 31951115 Free PMC article.
-
Reactive Oxygen Stimulation of Interleukin-6 Release in the Human Trophoblast Cell Line HTR-8/SVneo by the Trichlorethylene Metabolite S-(1,2-Dichloro)-l-Cysteine.Biol Reprod. 2016 Sep;95(3):66. doi: 10.1095/biolreprod.116.139261. Epub 2016 Aug 3. Biol Reprod. 2016. PMID: 27488030 Free PMC article.
-
Regulators involved in trophoblast syncytialization in the placenta of intrauterine growth restriction.Front Endocrinol (Lausanne). 2023 Jan 31;14:1107182. doi: 10.3389/fendo.2023.1107182. eCollection 2023. Front Endocrinol (Lausanne). 2023. PMID: 36798658 Free PMC article. Review.
References
-
- Wang R; Dang Y-L; Zheng R; Li Y; Li W; Lu X; Wang L-J; Zhu C; Lin H-Y; Wang H Live Cell Imaging of In Vitro Human Trophoblast Syncytialization1. Biol. Reprod 2014, 90, No. 117. - PubMed
-
- Pötgens A; Schmitz U; Bose P; Versmold A; Kaufmann P; Frank H-G Mechanisms of Syncytial Fusion: A Review. Placenta 2002, 23, S107–S113. - PubMed
-
- Wice B; Menton D; Geuze H; Schwartz AL Modulators of Cyclic AMP Metabolism Induce Syncytiotrophoblast Formation in Vitro. Exp. Cell Res 1990, 186, 306–16. - PubMed
-
- Cantle SJ; Kaufmann P; Luckhardt M; Schweikhart G Interpretation of Syncytial Sprouts and Bridges in the Human Placenta. Placenta 1987, 8, 2212–34. - PubMed
Publication types
MeSH terms
Substances
Grants and funding
LinkOut - more resources
Full Text Sources