Dual role of the foot-and-mouth disease virus 3B1 protein in the replication complex: As protein primer and as an essential component to recruit 3Dpol to membranes
- PMID: 37126532
- PMCID: PMC10174528
- DOI: 10.1371/journal.ppat.1011373
Dual role of the foot-and-mouth disease virus 3B1 protein in the replication complex: As protein primer and as an essential component to recruit 3Dpol to membranes
Abstract
Picornavirus genome replication takes place in specialized intracellular membrane compartments that concentrate viral RNA and proteins as well as a number of host factors that also participate in the process. The core enzyme in the replication machinery is the viral RNA-dependent RNA polymerase (RdRP) 3Dpol. Replication requires the primer protein 3B (or VPg) attached to two uridine molecules. 3B uridylylation is also catalysed by 3Dpol. Another critical interaction in picornavirus replication is that between 3Dpol and the precursor 3AB, a membrane-binding protein responsible for the localization of 3Dpol to the membranous compartments at which replication occurs. Unlike other picornaviruses, the animal pathogen foot-and-mouth disease virus (FMDV), encodes three non-identical copies of the 3B (3B1, 3B2, and 3B3) that could be specialized in different functions within the replication complex. Here, we have used a combination of biophysics, molecular and structural biology approaches to characterize the functional binding of FMDV 3B1 to the base of the palm of 3Dpol. The 1.7 Å resolution crystal structure of the FMDV 3Dpol -3B1 complex shows that 3B1 simultaneously links two 3Dpol molecules by binding at the bottom of their palm subdomains in an almost symmetric way. The two 3B1 contact surfaces involve a combination of hydrophobic and basic residues at the N- (G5-P6, R9; Region I) and C-terminus (R16, L19-P20; Region II) of this small protein. Enzyme-Linked Immunosorbent Assays (ELISA) show that the two 3B1 binding sites play a role in 3Dpol binding, with region II presenting the highest affinity. ELISA assays show that 3Dpol has higher binding affinity for 3B1 than for 3B2 or 3B3. Membrane-based pull-down assays show that 3B1 region II, and to a lesser extent also region I play essential roles in mediating the interaction of 3AB with the polymerase and its recruitment to intracellular membranes.
Copyright: © 2023 Ferrer-Orta et al. This is an open access article distributed under the terms of the Creative Commons Attribution License, which permits unrestricted use, distribution, and reproduction in any medium, provided the original author and source are credited.
Conflict of interest statement
The authors have declared that no competing interests exist.
Figures
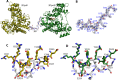
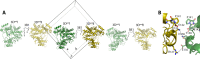
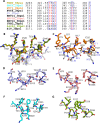
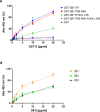
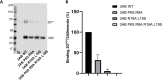
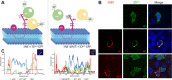
Similar articles
-
The multiple roles of viral 3Dpol protein in picornavirus infections.Virulence. 2024 Dec;15(1):2333562. doi: 10.1080/21505594.2024.2333562. Epub 2024 Apr 15. Virulence. 2024. PMID: 38622757 Free PMC article. Review.
-
Genetic economy in picornaviruses: Foot-and-mouth disease virus replication exploits alternative precursor cleavage pathways.PLoS Pathog. 2017 Oct 2;13(10):e1006666. doi: 10.1371/journal.ppat.1006666. eCollection 2017 Oct. PLoS Pathog. 2017. PMID: 28968463 Free PMC article.
-
Factors required for the Uridylylation of the foot-and-mouth disease virus 3B1, 3B2, and 3B3 peptides by the RNA-dependent RNA polymerase (3Dpol) in vitro.J Virol. 2005 Jun;79(12):7698-706. doi: 10.1128/JVI.79.12.7698-7706.2005. J Virol. 2005. PMID: 15919922 Free PMC article.
-
Both cis and trans Activities of Foot-and-Mouth Disease Virus 3D Polymerase Are Essential for Viral RNA Replication.J Virol. 2016 Jul 11;90(15):6864-6883. doi: 10.1128/JVI.00469-16. Print 2016 Aug 1. J Virol. 2016. PMID: 27194768 Free PMC article.
-
Structural insights into replication initiation and elongation processes by the FMDV RNA-dependent RNA polymerase.Curr Opin Struct Biol. 2009 Dec;19(6):752-8. doi: 10.1016/j.sbi.2009.10.016. Epub 2009 Nov 13. Curr Opin Struct Biol. 2009. PMID: 19914060 Review.
Cited by
-
The multiple roles of viral 3Dpol protein in picornavirus infections.Virulence. 2024 Dec;15(1):2333562. doi: 10.1080/21505594.2024.2333562. Epub 2024 Apr 15. Virulence. 2024. PMID: 38622757 Free PMC article. Review.
-
Multiple functions of the nonstructural protein 3D in picornavirus infection.Front Immunol. 2024 Apr 2;15:1365521. doi: 10.3389/fimmu.2024.1365521. eCollection 2024. Front Immunol. 2024. PMID: 38629064 Free PMC article. Review.
References
-
- Ehrenfeld Ellie.; Domingo Esteban.; Roos Raymond P., eds. (2010) The Picornvirus. 2010; ASM Press, Washington, DC.
-
- Wimmer E; Paul AV. The making of a picornavirus genome. In The Picornavirus; Ehrenfeld, Domingo E, E, Ross RP, Eds.; ASM Press: Washington, DC, USA, 2010; pp. 33–55.
Publication types
MeSH terms
Substances
Grants and funding
LinkOut - more resources
Full Text Sources
Research Materials