Dynamic Intestinal Stem Cell Plasticity and Lineage Remodeling by a Nutritional Environment Relevant to Human Risk for Tumorigenesis
- PMID: 37097719
- PMCID: PMC10390890
- DOI: 10.1158/1541-7786.MCR-22-1000
Dynamic Intestinal Stem Cell Plasticity and Lineage Remodeling by a Nutritional Environment Relevant to Human Risk for Tumorigenesis
Abstract
New Western-style diet 1 (NWD1), a purified diet establishing mouse exposure to key nutrients recapitulating levels that increase human risk for intestinal cancer, reproducibly causes mouse sporadic intestinal and colonic tumors reflecting human etiology, incidence, frequency, and lag with developmental age. Complex NWD1 stem cell and lineage reprogramming was deconvolved by bulk and single-cell RNA sequencing, single-cell Assay for Transposase-Accessible Chromatin using sequencing, functional genomics, and imaging. NWD1 extensively, rapidly, and reversibly, reprogrammed Lgr5hi stem cells, epigenetically downregulating Ppargc1a expression, altering mitochondrial structure and function. This suppressed Lgr5hi stem cell functions and developmental maturation of Lgr5hi cell progeny as cells progressed through progenitor cell compartments, recapitulated by Ppargc1a genetic inactivation in Lgr5hi cells in vivo. Mobilized Bmi1+, Ascl2hi cells adapted lineages to the nutritional environment and elevated antigen processing and presentation pathways, especially in mature enterocytes, causing chronic, protumorigenic low-level inflammation. There were multiple parallels between NWD1 remodeling of stem cells and lineages with pathogenic mechanisms in human inflammatory bowel disease, also protumorigenic. Moreover, the shift to alternate stem cells reflects that the balance between Lgr5-positive and -negative stem cells in supporting human colon tumors is determined by environmental influences. Stem cell and lineage plasticity in response to nutrients supports historic concepts of homeostasis as a continual adaptation to environment, with the human mucosa likely in constant flux in response to changing nutrient exposures.
Implications: Although oncogenic mutations provide a competitive advantage to intestinal epithelial cells in clonal expansion, the competition is on a playing field dynamically sculpted by the nutritional environment, influencing which cells dominate in mucosal maintenance and tumorigenesis.
©2023 The Authors; Published by the American Association for Cancer Research.
Figures
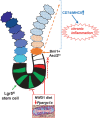
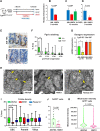
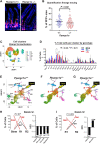
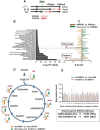
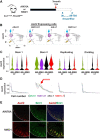
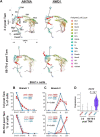
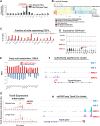
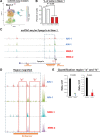
Comment in
- 1541-7786. doi: 10.1158/1541-7786.MCR-21-8-HI
Similar articles
-
Non-stem cell lineages as an alternative origin of intestinal tumorigenesis in the context of inflammation.Nat Genet. 2024 Jul;56(7):1456-1467. doi: 10.1038/s41588-024-01801-y. Epub 2024 Jun 20. Nat Genet. 2024. PMID: 38902475 Free PMC article.
-
Vitamin D and the nutritional environment in functions of intestinal stem cells: Implications for tumorigenesis and prevention.J Steroid Biochem Mol Biol. 2020 Apr;198:105556. doi: 10.1016/j.jsbmb.2019.105556. Epub 2019 Nov 26. J Steroid Biochem Mol Biol. 2020. PMID: 31783155 Free PMC article. Review.
-
The nutritional environment determines which and how intestinal stem cells contribute to homeostasis and tumorigenesis.Carcinogenesis. 2019 Aug 22;40(8):937-946. doi: 10.1093/carcin/bgz106. Carcinogenesis. 2019. PMID: 31169292 Free PMC article.
-
Environmental Impact on Intestinal Stem Cell Functions in Mucosal Homeostasis and Tumorigenesis.J Cell Biochem. 2017 May;118(5):943-952. doi: 10.1002/jcb.25719. Epub 2017 Jan 11. J Cell Biochem. 2017. PMID: 27584938 Free PMC article. Review.
-
BHLHA15-Positive Secretory Precursor Cells Can Give Rise to Tumors in Intestine and Colon in Mice.Gastroenterology. 2019 Mar;156(4):1066-1081.e16. doi: 10.1053/j.gastro.2018.11.024. Epub 2018 Nov 15. Gastroenterology. 2019. PMID: 30448068 Free PMC article.
Cited by
-
Intestinal stem cells: guardians of homeostasis in health and aging amid environmental challenges.Exp Mol Med. 2024 Mar;56(3):495-500. doi: 10.1038/s12276-024-01179-1. Epub 2024 Mar 1. Exp Mol Med. 2024. PMID: 38424189 Free PMC article. Review.
-
Heat-inactivated Bifidobacterium adolescentis ameliorates colon senescence through Paneth-like-cell-mediated stem cell activation.Nat Commun. 2023 Sep 30;14(1):6121. doi: 10.1038/s41467-023-41827-0. Nat Commun. 2023. PMID: 37777508 Free PMC article.
-
Dietary and metabolic effects on intestinal stem cells in health and disease.Nat Rev Gastroenterol Hepatol. 2025 Jan;22(1):23-38. doi: 10.1038/s41575-024-00980-7. Epub 2024 Oct 2. Nat Rev Gastroenterol Hepatol. 2025. PMID: 39358589 Review.
-
High-fat diet enhances cell proliferation and compromises intestinal permeability in a translational canine intestinal organoid model.BMC Mol Cell Biol. 2024 Apr 30;25(1):14. doi: 10.1186/s12860-024-00512-w. BMC Mol Cell Biol. 2024. PMID: 38689222 Free PMC article.
-
Non-stem cell lineages as an alternative origin of intestinal tumorigenesis in the context of inflammation.Nat Genet. 2024 Jul;56(7):1456-1467. doi: 10.1038/s41588-024-01801-y. Epub 2024 Jun 20. Nat Genet. 2024. PMID: 38902475 Free PMC article.
References
-
- Shivdasani RA. Race to the bottom: darwinian competition in early intestinal tumorigenesis. Cell Stem Cell 2021;28:1340–2. - PubMed
Publication types
MeSH terms
Grants and funding
LinkOut - more resources
Full Text Sources
Molecular Biology Databases