Brainstem neural mechanisms controlling locomotion with special reference to basal vertebrates
- PMID: 37063386
- PMCID: PMC10098025
- DOI: 10.3389/fncir.2023.910207
Brainstem neural mechanisms controlling locomotion with special reference to basal vertebrates
Abstract
Over the last 60 years, the basic neural circuitry responsible for the supraspinal control of locomotion has progressively been uncovered. Initially, significant progress was made in identifying the different supraspinal structures controlling locomotion in mammals as well as some of the underlying mechanisms. It became clear, however, that the complexity of the mammalian central nervous system (CNS) prevented researchers from characterizing the detailed cellular mechanisms involved and that animal models with a simpler nervous system were needed. Basal vertebrate species such as lampreys, xenopus embryos, and zebrafish became models of choice. More recently, optogenetic approaches have considerably revived interest in mammalian models. The mesencephalic locomotor region (MLR) is an important brainstem region known to control locomotion in all vertebrate species examined to date. It controls locomotion through intermediary cells in the hindbrain, the reticulospinal neurons (RSNs). The MLR comprises populations of cholinergic and glutamatergic neurons and their specific contribution to the control of locomotion is not fully resolved yet. Moreover, the downward projections from the MLR to RSNs is still not fully understood. Reporting on discoveries made in different animal models, this review article focuses on the MLR, its projections to RSNs, and the contribution of these neural elements to the control of locomotion. Excellent and detailed reviews on the brainstem control of locomotion have been recently published with emphasis on mammalian species. The present review article focuses on findings made in basal vertebrates such as the lamprey, to help direct new research in mammals, including humans.
Keywords: acetylcholine; descending control; glutamate; locomotion; mesencephalic locomotor region (MLR); neuromodulation.
Copyright © 2023 Lacroix-Ouellette and Dubuc.
Conflict of interest statement
The authors declare that the research was conducted in the absence of any commercial or financial relationships that could be construed as a potential conflict of interest.
Figures
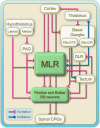
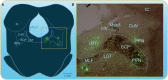
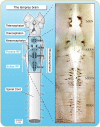
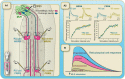
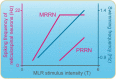
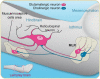
Similar articles
-
Initiation of locomotion in lampreys.Brain Res Rev. 2008 Jan;57(1):172-82. doi: 10.1016/j.brainresrev.2007.07.016. Epub 2007 Aug 22. Brain Res Rev. 2008. PMID: 17916380 Review.
-
A Brainstem Neural Substrate for Stopping Locomotion.J Neurosci. 2019 Feb 6;39(6):1044-1057. doi: 10.1523/JNEUROSCI.1992-18.2018. Epub 2018 Dec 12. J Neurosci. 2019. PMID: 30541913 Free PMC article.
-
Chapter 4--supraspinal control of locomotion: the mesencephalic locomotor region.Prog Brain Res. 2011;188:51-70. doi: 10.1016/B978-0-444-53825-3.00009-7. Prog Brain Res. 2011. PMID: 21333802 Review.
-
Cholinergic Modulation of Locomotor Circuits in Vertebrates.Int J Mol Sci. 2022 Sep 14;23(18):10738. doi: 10.3390/ijms231810738. Int J Mol Sci. 2022. PMID: 36142651 Free PMC article. Review.
-
The mesencephalic locomotor region recruits V2a reticulospinal neurons to drive forward locomotion in larval zebrafish.Nat Neurosci. 2023 Oct;26(10):1775-1790. doi: 10.1038/s41593-023-01418-0. Epub 2023 Sep 4. Nat Neurosci. 2023. PMID: 37667039 Free PMC article.
Cited by
-
Epidural Spinal Cord Stimulation for Spinal Cord Injury in Humans: A Systematic Review.J Clin Med. 2024 Feb 14;13(4):1090. doi: 10.3390/jcm13041090. J Clin Med. 2024. PMID: 38398403 Free PMC article. Review.
References
Publication types
MeSH terms
Grants and funding
LinkOut - more resources
Full Text Sources