Restraint of IFN-γ expression through a distal silencer CNS-28 for tissue homeostasis
- PMID: 37040761
- PMCID: PMC10175192
- DOI: 10.1016/j.immuni.2023.03.006
Restraint of IFN-γ expression through a distal silencer CNS-28 for tissue homeostasis
Abstract
Interferon-γ (IFN-γ) is a key cytokine in response to viral or intracellular bacterial infection in mammals. While a number of enhancers are described to promote IFN-γ responses, to the best of our knowledge, no silencers for the Ifng gene have been identified. By examining H3K4me1 histone modification in naive CD4+ T cells within Ifng locus, we identified a silencer (CNS-28) that restrains Ifng expression. Mechanistically, CNS-28 maintains Ifng silence by diminishing enhancer-promoter interactions within Ifng locus in a GATA3-dependent but T-bet-independent manner. Functionally, CNS-28 restrains Ifng transcription in NK cells, CD4+ cells, and CD8+ T cells during both innate and adaptive immune responses. Moreover, CNS-28 deficiency resulted in repressed type 2 responses due to elevated IFN-γ expression, shifting Th1 and Th2 paradigm. Thus, CNS-28 activity ensures immune cell quiescence by cooperating with other regulatory cis elements within the Ifng gene locus to minimize autoimmunity.
Keywords: IFN-gamma expression; chromatin interaction; distal enhancer; distal repressor of transcription; tissue homeostasis.
Published by Elsevier Inc.
Conflict of interest statement
Declaration of interests The authors declare no competing interests.
Figures
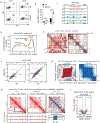
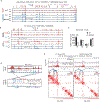
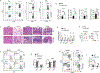
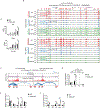
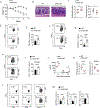
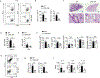
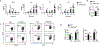
Comment in
-
To loop or not to loop? CNS-28 is the answer.Immunity. 2023 May 9;56(5):895-897. doi: 10.1016/j.immuni.2023.04.009. Immunity. 2023. PMID: 37163987
Similar articles
-
Diverse functions of distal regulatory elements at the IFNG locus.J Immunol. 2012 Feb 15;188(4):1726-33. doi: 10.4049/jimmunol.1102879. Epub 2012 Jan 13. J Immunol. 2012. PMID: 22246629 Free PMC article.
-
Loss of methylation at the IFNG promoter and CNS-1 is associated with the development of functional IFN-γ memory in human CD4(+) T lymphocytes.Eur J Immunol. 2013 Mar;43(3):793-804. doi: 10.1002/eji.201242858. Eur J Immunol. 2013. PMID: 23255246
-
Deletion of a conserved cis-element in the Ifng locus highlights the role of acute histone acetylation in modulating inducible gene transcription.PLoS Genet. 2014 Jan;10(1):e1003969. doi: 10.1371/journal.pgen.1003969. Epub 2014 Jan 9. PLoS Genet. 2014. PMID: 24415943 Free PMC article.
-
Regulation of interferon-gamma during innate and adaptive immune responses.Adv Immunol. 2007;96:41-101. doi: 10.1016/S0065-2776(07)96002-2. Adv Immunol. 2007. PMID: 17981204 Review.
-
A cellular and molecular view of T helper 17 cell plasticity in autoimmunity.J Autoimmun. 2018 Feb;87:1-15. doi: 10.1016/j.jaut.2017.12.007. Epub 2017 Dec 22. J Autoimmun. 2018. PMID: 29275836 Review.
Cited by
-
Interferon signaling and ferroptosis in tumor immunology and therapy.NPJ Precis Oncol. 2024 Aug 10;8(1):177. doi: 10.1038/s41698-024-00668-w. NPJ Precis Oncol. 2024. PMID: 39127858 Free PMC article.
-
Dysregulation in IFN-γ signaling and response: the barricade to tumor immunotherapy.Front Immunol. 2023 May 18;14:1190333. doi: 10.3389/fimmu.2023.1190333. eCollection 2023. Front Immunol. 2023. PMID: 37275859 Free PMC article. Review.
-
Regulation of T helper cell differentiation by the interplay between histone modification and chromatin interaction.Immunity. 2024 May 14;57(5):987-1004.e5. doi: 10.1016/j.immuni.2024.03.018. Epub 2024 Apr 12. Immunity. 2024. PMID: 38614090
-
KMT2D regulates activation, localization, and integrin expression by T-cells.Front Immunol. 2024 May 3;15:1341745. doi: 10.3389/fimmu.2024.1341745. eCollection 2024. Front Immunol. 2024. PMID: 38765012 Free PMC article.
-
Three-dimensional chromatin reorganization regulates B cell development during ageing.Nat Cell Biol. 2024 Jun;26(6):991-1002. doi: 10.1038/s41556-024-01424-9. Epub 2024 Jun 12. Nat Cell Biol. 2024. PMID: 38866970 Free PMC article.
References
Publication types
MeSH terms
Substances
Grants and funding
LinkOut - more resources
Full Text Sources
Other Literature Sources
Molecular Biology Databases
Research Materials