Identification of key residues of the DNA glycosylase OGG1 controlling efficient DNA sampling and recruitment to oxidized bases in living cells
- PMID: 37021552
- PMCID: PMC10250219
- DOI: 10.1093/nar/gkad243
Identification of key residues of the DNA glycosylase OGG1 controlling efficient DNA sampling and recruitment to oxidized bases in living cells
Abstract
The DNA-glycosylase OGG1 oversees the detection and clearance of the 7,8-dihydro-8-oxoguanine (8-oxoG), which is the most frequent form of oxidized base in the genome. This lesion is deeply buried within the double-helix and its detection requires careful inspection of the bases by OGG1 via a mechanism that remains only partially understood. By analyzing OGG1 dynamics in the nucleus of living human cells, we demonstrate that the glycosylase constantly samples the DNA by rapidly alternating between diffusion within the nucleoplasm and short transits on the DNA. This sampling process, that we find to be tightly regulated by the conserved residue G245, is crucial for the rapid recruitment of OGG1 at oxidative lesions induced by laser micro-irradiation. Furthermore, we show that residues Y203, N149 and N150, while being all involved in early stages of 8-oxoG probing by OGG1 based on previous structural data, differentially regulate the sampling of the DNA and recruitment to oxidative lesions.
© The Author(s) 2023. Published by Oxford University Press on behalf of Nucleic Acids Research.
Figures
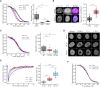
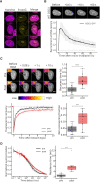
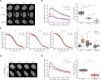
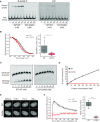
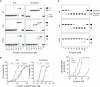
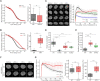
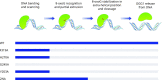
Similar articles
-
Spatio-temporal dynamics of the DNA glycosylase OGG1 in finding and processing 8-oxoguanine.DNA Repair (Amst). 2023 Sep;129:103550. doi: 10.1016/j.dnarep.2023.103550. Epub 2023 Jul 31. DNA Repair (Amst). 2023. PMID: 37542751
-
Lost in the Crowd: How Does Human 8-Oxoguanine DNA Glycosylase 1 (OGG1) Find 8-Oxoguanine in the Genome?Int J Mol Sci. 2020 Nov 7;21(21):8360. doi: 10.3390/ijms21218360. Int J Mol Sci. 2020. PMID: 33171795 Free PMC article. Review.
-
Quantifying Activity for Repair of the DNA Lesion 8-Oxoguanine by Oxoguanine Glycosylase 1 (OGG1) in Mouse Adult and Fetal Brain Nuclear Extracts Using Biotin-Labeled DNA.Methods Mol Biol. 2019;1965:329-349. doi: 10.1007/978-1-4939-9182-2_22. Methods Mol Biol. 2019. PMID: 31069685
-
Oxidized base 8-oxoguanine, a product of DNA repair processes, contributes to dendritic cell activation.Free Radic Biol Med. 2019 Nov 1;143:209-220. doi: 10.1016/j.freeradbiomed.2019.08.010. Epub 2019 Aug 10. Free Radic Biol Med. 2019. PMID: 31408726 Free PMC article.
-
Repair of 8-oxo-7,8-dihydroguanine in prokaryotic and eukaryotic cells: Properties and biological roles of the Fpg and OGG1 DNA N-glycosylases.Free Radic Biol Med. 2017 Jun;107:179-201. doi: 10.1016/j.freeradbiomed.2016.11.042. Epub 2016 Nov 27. Free Radic Biol Med. 2017. PMID: 27903453 Review.
Cited by
-
Human 8-oxoguanine glycosylase OGG1 binds nucleosome at the dsDNA ends and the super-helical locations.Commun Biol. 2024 Sep 28;7(1):1202. doi: 10.1038/s42003-024-06919-7. Commun Biol. 2024. PMID: 39341999 Free PMC article.
-
Multi-Scale Imaging of the Dynamic Organization of Chromatin.Int J Mol Sci. 2023 Nov 4;24(21):15975. doi: 10.3390/ijms242115975. Int J Mol Sci. 2023. PMID: 37958958 Free PMC article. Review.
-
Polymorphisms of DNA Repair Genes in Thyroid Cancer.Int J Mol Sci. 2024 May 30;25(11):5995. doi: 10.3390/ijms25115995. Int J Mol Sci. 2024. PMID: 38892180 Free PMC article. Review.
References
-
- Hall D.B., Holmlin R.E., Barton J.K.. Oxidative DNA damage through long-range electron transfer. Nature. 1996; 382:731–735. - PubMed
Publication types
MeSH terms
Substances
LinkOut - more resources
Full Text Sources
Research Materials