An IL-6/STAT3/MR/FGF21 axis mediates heart-liver cross-talk after myocardial infarction
- PMID: 37018396
- PMCID: PMC10075967
- DOI: 10.1126/sciadv.ade4110
An IL-6/STAT3/MR/FGF21 axis mediates heart-liver cross-talk after myocardial infarction
Abstract
The liver plays a protective role in myocardial infarction (MI). However, very little is known about the mechanisms. Here, we identify mineralocorticoid receptor (MR) as a pivotal nexus that conveys communications between the liver and the heart during MI. Hepatocyte MR deficiency and MR antagonist spironolactone both improve cardiac repair after MI through regulation on hepatic fibroblast growth factor 21 (FGF21), illustrating an MR/FGF21 axis that underlies the liver-to-heart protection against MI. In addition, an upstreaming acute interleukin-6 (IL-6)/signal transducer and activator of transcription 3 (STAT3) pathway transmits the heart-to-liver signal to suppress MR expression after MI. Hepatocyte Il6 receptor deficiency and Stat3 deficiency both aggravate cardiac injury through their regulation on the MR/FGF21 axis. Therefore, we have unveiled an IL-6/STAT3/MR/FGF21 signaling axis that mediates heart-liver cross-talk during MI. Targeting the signaling axis and the cross-talk could provide new strategies to treat MI and heart failure.
Figures
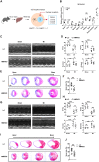
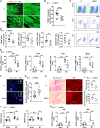
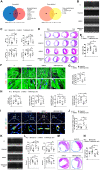
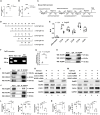
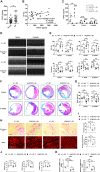
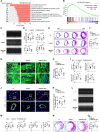
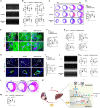
Similar articles
-
Liver-heart crosstalk controls IL-22 activity in cardiac protection after myocardial infarction.Theranostics. 2018 Aug 10;8(16):4552-4562. doi: 10.7150/thno.24723. eCollection 2018. Theranostics. 2018. PMID: 30214638 Free PMC article.
-
Mineralocorticoid Receptor Blocker Prevents Mineralocorticoid Receptor-Mediated Inflammation by Modulating Transcriptional Activity of Mineralocorticoid Receptor-p65-Signal Transducer and Activator of Transcription 3 Complex.J Am Heart Assoc. 2024 Sep 17;13(18):e030941. doi: 10.1161/JAHA.123.030941. Epub 2024 Sep 9. J Am Heart Assoc. 2024. PMID: 39248263
-
Therapeutic activation of signal transducer and activator of transcription 3 by interleukin-11 ameliorates cardiac fibrosis after myocardial infarction.Circulation. 2010 Feb 9;121(5):684-91. doi: 10.1161/CIRCULATIONAHA.109.893677. Epub 2010 Jan 25. Circulation. 2010. PMID: 20100971
-
Activating the interleukin-6-Gp130-STAT3 pathway ameliorates ventricular electrical stability in myocardial infarction rats by modulating neurotransmitters in the paraventricular nucleus.BMC Cardiovasc Disord. 2020 Feb 5;20(1):60. doi: 10.1186/s12872-020-01363-x. BMC Cardiovasc Disord. 2020. PMID: 32024466 Free PMC article.
-
The myocardial JAK/STAT pathway: from protection to failure.Pharmacol Ther. 2008 Nov;120(2):172-85. doi: 10.1016/j.pharmthera.2008.08.002. Epub 2008 Aug 23. Pharmacol Ther. 2008. PMID: 18786563 Review.
Cited by
-
Fibroblast Growth Factor 21 Suppressed Neutrophil Extracellular Traps Induced by Myocardial Ischemia/Reperfusion Injury via Adenosine Monophosphate-Activated Protein Kinase.Cardiol Res. 2024 Oct;15(5):404-414. doi: 10.14740/cr1705. Epub 2024 Oct 11. Cardiol Res. 2024. PMID: 39420979 Free PMC article.
-
The Gut Microbiota Mediates the Protective Effects of Spironolactone on Myocardial Infarction.J Microbiol. 2024 Sep 3. doi: 10.1007/s12275-024-00164-7. Online ahead of print. J Microbiol. 2024. PMID: 39225943
-
Liver regeneration after injury: Mechanisms, cellular interactions and therapeutic innovations.Clin Transl Med. 2024 Aug;14(8):e1812. doi: 10.1002/ctm2.1812. Clin Transl Med. 2024. PMID: 39152680 Free PMC article. Review.
-
Histones Methyltransferase NSD3 Inhibits Lung Adenocarcinoma Glycolysis Through Interacting with PPP1CB to Decrease STAT3 Signaling Pathway.Adv Sci (Weinh). 2024 Oct;11(38):e2400381. doi: 10.1002/advs.202400381. Epub 2024 Aug 9. Adv Sci (Weinh). 2024. PMID: 39119928 Free PMC article.
-
A novel mouse model of hepatocyte-specific apoptosis-induced myeloid cell-dominant sterile liver injury and repair response.Am J Physiol Gastrointest Liver Physiol. 2024 Oct 1;327(4):G499-G512. doi: 10.1152/ajpgi.00005.2024. Epub 2024 Aug 6. Am J Physiol Gastrointest Liver Physiol. 2024. PMID: 39104322
References
-
- Bernardo B. C., Ooi J. Y. Y., Weeks K. L., Patterson N. L., McMullen J. R., Understanding key mechanisms of exercise-induced cardiac protection to mitigate disease: Current knowledge and emerging concepts. Physiol. Rev. 98, 419–475 (2018). - PubMed
-
- Khanlou H., Souto H., Lippmann M., Muñoz S., Rothstein K., Ozden Z., Resolution of adult respiratory distress syndrome after recovery from fulminant hepatic failure. Am. J. Med. Sci. 317, 134–136 (1999). - PubMed
-
- Ali M., Wall W. J., Resolution of the adult respiratory distress syndrome following colectomy and liver transplantation. Chest 98, 1032–1034 (1990). - PubMed
MeSH terms
Substances
LinkOut - more resources
Full Text Sources
Other Literature Sources
Medical
Molecular Biology Databases
Miscellaneous