Development of a Synthetic, Injectable Hydrogel to Capture Residual Glioblastoma and Glioblastoma Stem-Like Cells with CXCL12-Mediated Chemotaxis
- PMID: 37014179
- PMCID: PMC11469263
- DOI: 10.1002/adhm.202300671
Development of a Synthetic, Injectable Hydrogel to Capture Residual Glioblastoma and Glioblastoma Stem-Like Cells with CXCL12-Mediated Chemotaxis
Abstract
Glioblastoma (GBM), characterized by high infiltrative capacity, is the most common and deadly type of primary brain tumor in adults. GBM cells, including therapy-resistant glioblastoma stem-like cells (GSCs), invade the healthy brain parenchyma to form secondary tumors even after patients undergo surgical resection and chemoradiotherapy. New techniques are therefore urgently needed to eradicate these residual tumor cells. A thiol-Michael addition injectable hydrogel for compatibility with GBM therapy is previously characterized and optimized. This study aims to develop the hydrogel further to capture GBM/GSCs through CXCL12-mediated chemotaxis. The release kinetics of hydrogel payloads are investigated, migration and invasion assays in response to chemoattractants are performed, and the GBM-hydrogel interactions in vitro are studied. With a novel dual-layer hydrogel platform, it is demonstrated that CXCL12 released from the synthetic hydrogel can induce the migration of U251 GBM cells and GSCs from the extracellular matrix microenvironment and promote invasion into the synthetic hydrogel via amoeboid migration. The survival of GBM cells entrapped deep into the synthetic hydrogel is limited, while live cells near the surface reinforce the hydrogel through fibronectin deposition. This synthetic hydrogel, therefore, demonstrates a promising method to attract and capture migratory GBM cells and GSCs responsive to CXCL12 chemotaxis.
Keywords: CXCL12 chemotaxis; cell migration; collagen-hyaluronic acid hydrogel; controlled delivery; glioblastoma cells; glioblastoma stem-like cells; thiol-Michael addition hydrogel.
© 2023 The Authors. Advanced Healthcare Materials published by Wiley-VCH GmbH.
Conflict of interest statement
Z.M.K., S.S.V., T.E.L., and E.V. are inventors on a pending patent related to this work. The authors declare no other financial or commercial conflicts of interest.
Figures
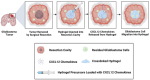
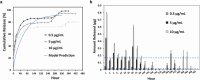
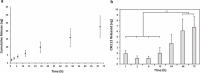
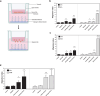
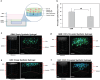
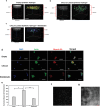
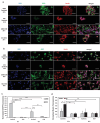
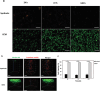
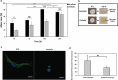
Similar articles
-
Development of an Injectable Hydrogel for Histotripsy Ablation Toward Future Glioblastoma Therapy Applications.Ann Biomed Eng. 2024 Dec;52(12):3157-3171. doi: 10.1007/s10439-024-03601-1. Epub 2024 Aug 30. Ann Biomed Eng. 2024. PMID: 39210157 Free PMC article.
-
Characterization and structure-property relationships of an injectable thiol-Michael addition hydrogel toward compatibility with glioblastoma therapy.Acta Biomater. 2022 May;144:266-278. doi: 10.1016/j.actbio.2022.03.016. Epub 2022 Mar 14. Acta Biomater. 2022. PMID: 35296443
-
Adult mouse subventricular zones stimulate glioblastoma stem cells specific invasion through CXCL12/CXCR4 signaling.Neuro Oncol. 2015 Jan;17(1):81-94. doi: 10.1093/neuonc/nou144. Epub 2014 Aug 1. Neuro Oncol. 2015. PMID: 25085362 Free PMC article.
-
Chemoresistance in high-grade gliomas: relevance of adenosine signalling in stem-like cells of glioblastoma multiforme.Curr Drug Targets. 2014;15(10):931-42. Curr Drug Targets. 2014. PMID: 25174341 Review.
-
Biomimetic models to examine microenvironmental regulation of glioblastoma stem cells.Cancer Lett. 2018 Aug 10;429:41-53. doi: 10.1016/j.canlet.2018.05.007. Epub 2018 May 8. Cancer Lett. 2018. PMID: 29746930 Review.
Cited by
-
Factors That Influence Base-Catalyzed Thiol-Ene Hydrogel Synthesis.Gels. 2023 Nov 20;9(11):917. doi: 10.3390/gels9110917. Gels. 2023. PMID: 37999007 Free PMC article.
-
Macroporous chitosan/alginate hydrogels crosslinked with genipin accumulate and retain glioblastoma cancer cells.RSC Adv. 2024 Nov 5;14(48):35286-35304. doi: 10.1039/d4ra06197g. eCollection 2024 Nov 4. RSC Adv. 2024. PMID: 39502176 Free PMC article.
-
Development of an Injectable Hydrogel for Histotripsy Ablation Toward Future Glioblastoma Therapy Applications.Ann Biomed Eng. 2024 Dec;52(12):3157-3171. doi: 10.1007/s10439-024-03601-1. Epub 2024 Aug 30. Ann Biomed Eng. 2024. PMID: 39210157 Free PMC article.
References
-
- Stupp R., Brada M., van den Bent M. J., Tonn J. C., Pentheroudakis G., Ann. Oncol. 2014, 25, iii93. - PubMed
Publication types
MeSH terms
Substances
LinkOut - more resources
Full Text Sources
Medical