Molecular Dynamics Simulations of the Proteins Regulating Synaptic Vesicle Fusion
- PMID: 36984694
- PMCID: PMC10058449
- DOI: 10.3390/membranes13030307
Molecular Dynamics Simulations of the Proteins Regulating Synaptic Vesicle Fusion
Abstract
Neuronal transmitters are packaged in synaptic vesicles (SVs) and released by the fusion of SVs with the presynaptic membrane (PM). An inflow of Ca2+ into the nerve terminal triggers fusion, and the SV-associated protein Synaptotagmin 1 (Syt1) serves as a Ca2+ sensor. In preparation for fusion, SVs become attached to the PM by the SNARE protein complex, a coiled-coil bundle that exerts the force overcoming SV-PM repulsion. A cytosolic protein Complexin (Cpx) attaches to the SNARE complex and differentially regulates the evoked and spontaneous release components. It is still debated how the dynamic interactions of Syt1, SNARE proteins and Cpx lead to fusion. This problem is confounded by heterogeneity in the conformational states of the prefusion protein-lipid complex and by the lack of tools to experimentally monitor the rapid conformational transitions of the complex, which occur at a sub-millisecond scale. However, these complications can be overcome employing molecular dynamics (MDs), a computational approach that enables simulating interactions and conformational transitions of proteins and lipids. This review discusses the use of molecular dynamics for the investigation of the pre-fusion protein-lipid complex. We discuss the dynamics of the SNARE complex between lipid bilayers, as well as the interactions of Syt1 with lipids and SNARE proteins, and Cpx regulating the assembly of the SNARE complex.
Keywords: SNARE complex; complexin; exocytosis; lipid bilayers; neuronal transmitters; synaptotagmin.
Conflict of interest statement
The authors declare no conflict of interest.
Figures
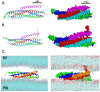
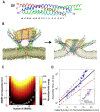
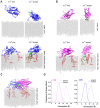
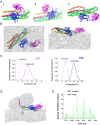
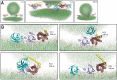
Similar articles
-
Dynamic formation of the protein-lipid prefusion complex.Biophys J. 2024 Oct 15;123(20):3569-3586. doi: 10.1016/j.bpj.2024.09.009. Epub 2024 Sep 10. Biophys J. 2024. PMID: 39257001
-
Dynamic Formation of the Protein-Lipid Pre-fusion Complex.bioRxiv [Preprint]. 2024 May 11:2024.04.17.589983. doi: 10.1101/2024.04.17.589983. bioRxiv. 2024. Update in: Biophys J. 2024 Oct 15;123(20):3569-3586. doi: 10.1016/j.bpj.2024.09.009 PMID: 38659925 Free PMC article. Updated. Preprint.
-
SNARE complex alters the interactions of the Ca2+ sensor synaptotagmin 1 with lipid bilayers.Biophys J. 2021 Feb 16;120(4):642-661. doi: 10.1016/j.bpj.2020.12.025. Epub 2021 Jan 14. Biophys J. 2021. PMID: 33453271 Free PMC article.
-
Models of synaptotagmin-1 to trigger Ca2+ -dependent vesicle fusion.FEBS Lett. 2018 Nov;592(21):3480-3492. doi: 10.1002/1873-3468.13193. Epub 2018 Jul 30. FEBS Lett. 2018. PMID: 30004579 Review.
-
Exocytosis and synaptic vesicle function.Compr Physiol. 2014 Jan;4(1):149-75. doi: 10.1002/cphy.c130021. Compr Physiol. 2014. PMID: 24692137 Review.
Cited by
-
Studying the Effects of Dissolved Noble Gases and High Hydrostatic Pressure on the Spherical DOPC Bilayer Using Molecular Dynamic Simulations.Membranes (Basel). 2024 Apr 12;14(4):89. doi: 10.3390/membranes14040089. Membranes (Basel). 2024. PMID: 38668117 Free PMC article.
-
Membrane Proteins: Function, Structure, and Dynamics.Membranes (Basel). 2023 Dec 9;13(12):904. doi: 10.3390/membranes13120904. Membranes (Basel). 2023. PMID: 38132908 Free PMC article.
References
Publication types
Grants and funding
LinkOut - more resources
Full Text Sources
Research Materials
Miscellaneous