The LINC Complex Inhibits Excessive Chromatin Repression
- PMID: 36980273
- PMCID: PMC10047284
- DOI: 10.3390/cells12060932
The LINC Complex Inhibits Excessive Chromatin Repression
Abstract
The Linker of Nucleoskeleton and Cytoskeleton (LINC) complex transduces nuclear mechanical inputs suggested to control chromatin organization and gene expression; however, the underlying mechanism is currently unclear. We show here that the LINC complex is needed to minimize chromatin repression in muscle tissue, where the nuclei are exposed to significant mechanical inputs during muscle contraction. To this end, the genomic binding profiles of Polycomb, Heterochromatin Protein1 (HP1a) repressors, and of RNA-Pol II were studied in Drosophila larval muscles lacking functional LINC complex. A significant increase in the binding of Polycomb and parallel reduction of RNA-Pol-II binding to a set of muscle genes was observed. Consistently, enhanced tri-methylated H3K9 and H3K27 repressive modifications and reduced chromatin activation by H3K9 acetylation were found. Furthermore, larger tri-methylated H3K27me3 repressive clusters, and chromatin redistribution from the nuclear periphery towards nuclear center, were detected in live LINC mutant larval muscles. Computer simulation indicated that the observed dissociation of the chromatin from the nuclear envelope promotes growth of tri-methylated H3K27 repressive clusters. Thus, we suggest that by promoting chromatin-nuclear envelope binding, the LINC complex restricts the size of repressive H3K27 tri-methylated clusters, thereby limiting the binding of Polycomb transcription repressor, directing robust transcription in muscle fibers.
Keywords: LINC complex; chromatin repression; epigenetics; muscle; nuclear mechanobiology.
Conflict of interest statement
The authors declare no conflict of interest.
Figures
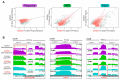
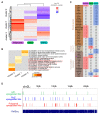
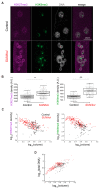
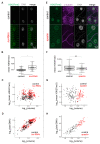
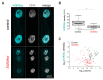
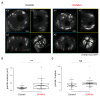
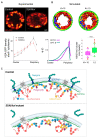
Similar articles
-
Polycomb proteins translate histone methylation to chromatin folding.J Biol Chem. 2023 Sep;299(9):105080. doi: 10.1016/j.jbc.2023.105080. Epub 2023 Jul 25. J Biol Chem. 2023. PMID: 37499944 Free PMC article.
-
Mechanotransduction via the LINC complex regulates DNA replication in myonuclei.J Cell Biol. 2018 Jun 4;217(6):2005-2018. doi: 10.1083/jcb.201708137. Epub 2018 Apr 12. J Cell Biol. 2018. PMID: 29650775 Free PMC article.
-
The trithorax group proteins Kismet and ASH1 promote H3K36 dimethylation to counteract Polycomb group repression in Drosophila.Development. 2013 Oct;140(20):4182-92. doi: 10.1242/dev.095786. Epub 2013 Sep 4. Development. 2013. PMID: 24004944 Free PMC article.
-
The plant LINC complex at the nuclear envelope.Chromosome Res. 2014 Jun;22(2):241-52. doi: 10.1007/s10577-014-9419-7. Chromosome Res. 2014. PMID: 24801343 Review.
-
Epigenetic Regulation by Polycomb Complexes from Drosophila to Human and Its Relation to Communicable Disease Pathogenesis.Int J Mol Sci. 2022 Oct 14;23(20):12285. doi: 10.3390/ijms232012285. Int J Mol Sci. 2022. PMID: 36293135 Free PMC article. Review.
Cited by
-
Data-Driven and Cell-Specific Determination of Nuclei-Associated Actin Structure.Small Struct. 2024 May;5(5):2300204. doi: 10.1002/sstr.202300204. Epub 2024 Feb 16. Small Struct. 2024. PMID: 39220563
-
Anchorage of H3K9-methylated heterochromatin to the nuclear periphery helps mediate P-cell nuclear migration though constricted spaces in Caenorhabditis elegans.bioRxiv [Preprint]. 2024 May 23:2024.05.22.595380. doi: 10.1101/2024.05.22.595380. bioRxiv. 2024. PMID: 38826247 Free PMC article. Preprint.
-
Histone Modification of Osteogenesis Related Genes Triggered by Substrate Topography Promotes Human Mesenchymal Stem Cell Differentiation.ACS Appl Mater Interfaces. 2023 Jun 28;15(25):29752-29766. doi: 10.1021/acsami.3c01481. Epub 2023 Jun 13. ACS Appl Mater Interfaces. 2023. PMID: 37310722 Free PMC article.
References
Publication types
MeSH terms
Substances
Grants and funding
LinkOut - more resources
Full Text Sources
Molecular Biology Databases